- Random article
- Teaching guide
- Privacy & cookies
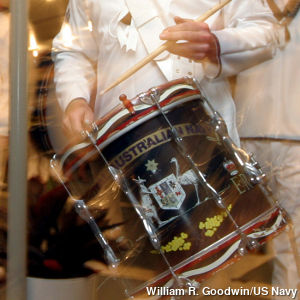
by Chris Woodford . Last updated: July 23, 2023.
Photo: Sound is energy we hear made by things that vibrate. Photo by William R. Goodwin courtesy of US Navy and Wikimedia Commons .

What is sound?
Photo: Sensing with sound: Light doesn't travel well through ocean water: over half the light falling on the sea surface is absorbed within the first meter of water; 100m down and only 1 percent of the surface light remains. That's largely why mighty creatures of the deep rely on sound for communication and navigation. Whales, famously, "talk" to one another across entire ocean basins, while dolphins use sound, like bats, for echolocation. Photo by Bill Thompson courtesy of US Fish and Wildlife Service .
Robert Boyle's classic experiment
Artwork: Robert Boyle's famous experiment with an alarm clock.
How sound travels
Artwork: Sound waves and ocean waves compared. Top: Sound waves are longitudinal waves: the air moves back and forth along the same line as the wave travels, making alternate patterns of compressions and rarefactions. Bottom: Ocean waves are transverse waves: the water moves back and forth at right angles to the line in which the wave travels.
The science of sound waves
Picture: Reflected sound is extremely useful for "seeing" underwater where light doesn't really travel—that's the basic idea behind sonar. Here's a side-scan sonar (reflected sound) image of a World War II boat wrecked on the seabed. Photo courtesy of U.S. National Oceanographic and Atmospheric Administration, US Navy, and Wikimedia Commons .
Whispering galleries and amphitheaters
Photos by Carol M. Highsmith: 1) The Capitol in Washington, DC has a whispering gallery inside its dome. Photo credit: The George F. Landegger Collection of District of Columbia Photographs in Carol M. Highsmith's America, Library of Congress , Prints and Photographs Division. 2) It's easy to hear people talking in the curved memorial amphitheater building at Arlington National Cemetery, Arlington, Virginia. Photo credit: Photographs in the Carol M. Highsmith Archive, Library of Congress , Prints and Photographs Division.
Measuring waves
Understanding amplitude and frequency, why instruments sound different, the speed of sound.
Photo: Breaking through the sound barrier creates a sonic boom. The mist you can see, which is called a condensation cloud, isn't necessarily caused by an aircraft flying supersonic: it can occur at lower speeds too. It happens because moist air condenses due to the shock waves created by the plane. You might expect the plane to compress the air as it slices through. But the shock waves it generates alternately expand and contract the air, producing both compressions and rarefactions. The rarefactions cause very low pressure and it's these that make moisture in the air condense, producing the cloud you see here. Photo by John Gay courtesy of US Navy and Wikimedia Commons .
Why does sound go faster in some things than in others?
Chart: Generally, sound travels faster in solids (right) than in liquids (middle) or gases (left)... but there are exceptions!
How to measure the speed of sound
Sound in practice, if you liked this article..., find out more, on this website.
- Electric guitars
- Speech synthesis
- Synthesizers
On other sites
- Explore Sound : A comprehensive educational site from the Acoustical Society of America, with activities for students of all ages.
- Sound Waves : A great collection of interactive science lessons from the University of Salford, which explains what sound waves are and the different ways in which they behave.
Educational books for younger readers
- Sound (Science in a Flash) by Georgia Amson-Bradshaw. Franklin Watts/Hachette, 2020. Simple facts, experiments, and quizzes fill this book; the visually exciting design will appeal to reluctant readers. Also for ages 7–9.
- Sound by Angela Royston. Raintree, 2017. A basic introduction to sound and musical sounds, including simple activities. Ages 7–9.
- Experimenting with Sound Science Projects by Robert Gardner. Enslow Publishers, 2013. A comprehensive 120-page introduction, running through the science of sound in some detail, with plenty of hands-on projects and activities (including welcome coverage of how to run controlled experiments using the scientific method). Ages 9–12.
- Cool Science: Experiments with Sound and Hearing by Chris Woodford. Gareth Stevens Inc, 2010. One of my own books, this is a short introduction to sound through practical activities, for ages 9–12.
- Adventures in Sound with Max Axiom, Super Scientist by Emily Sohn. Capstone, 2007. The original, graphic novel (comic book) format should appeal to reluctant readers. Ages 8–10.
Popular science
- The Sound Book: The Science of the Sonic Wonders of the World by Trevor Cox. W. W. Norton, 2014. An entertaining tour through everyday sound science.
Academic books
- Master Handbook of Acoustics by F. Alton Everest and Ken Pohlmann. McGraw-Hill Education, 2015. A comprehensive reference for undergraduates and sound-design professionals.
- The Science of Sound by Thomas D. Rossing, Paul A. Wheeler, and F. Richard Moore. Pearson, 2013. One of the most popular general undergraduate texts.
Text copyright © Chris Woodford 2009, 2021. All rights reserved. Full copyright notice and terms of use .
Rate this page
Tell your friends, cite this page, more to explore on our website....
- Get the book
- Send feedback
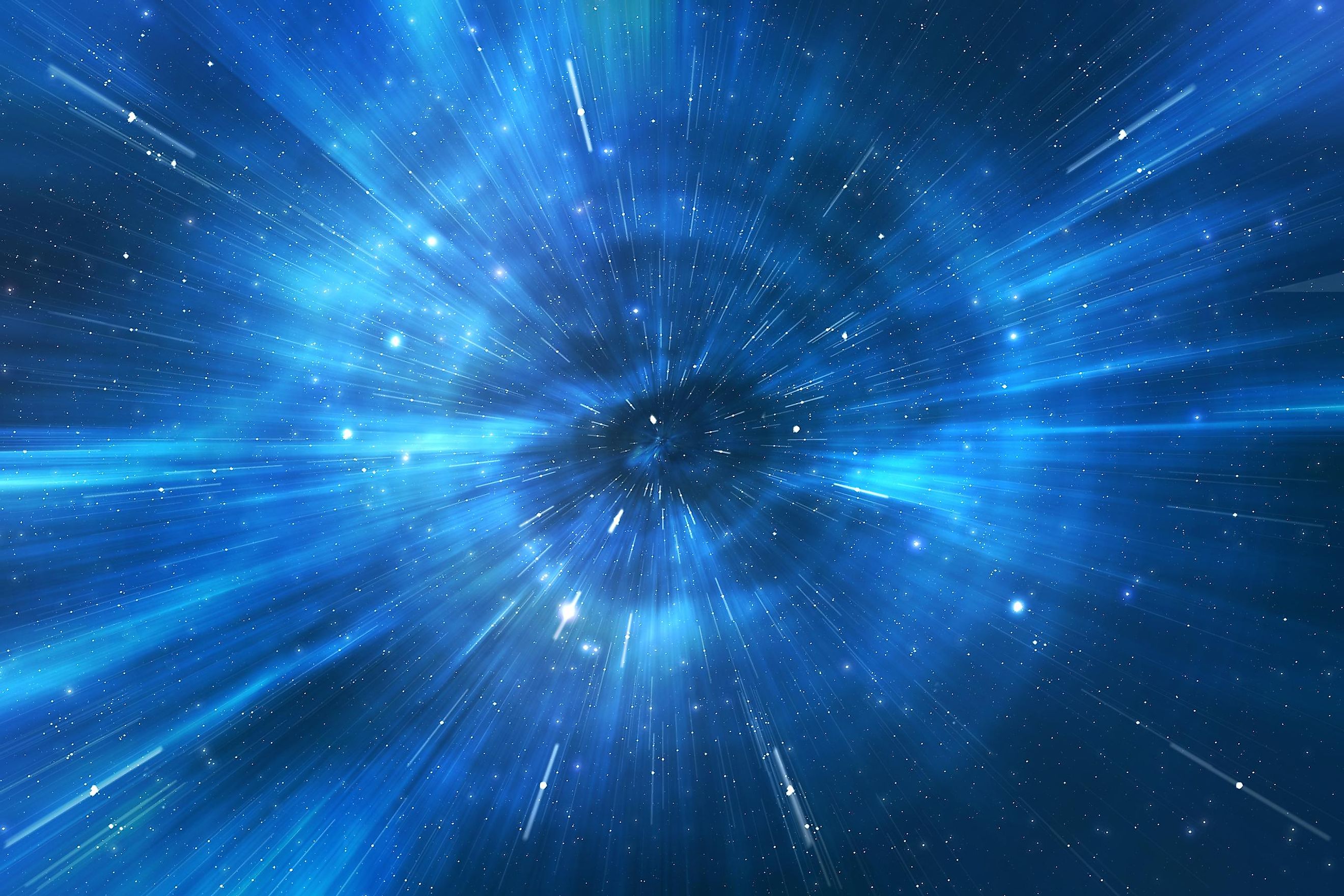
- Speed Of Sound vs Speed Of Light
The speed of sound and the speed of light are two common concepts that most of us likely learn about at some point in life. Both are defined rather simply, with the speed of sound being the speed that sound travels, and the speed of light being the speed at which light travels. Although these two concepts may sound similar, they are in fact radically different from one another. What are some of the differences between them?
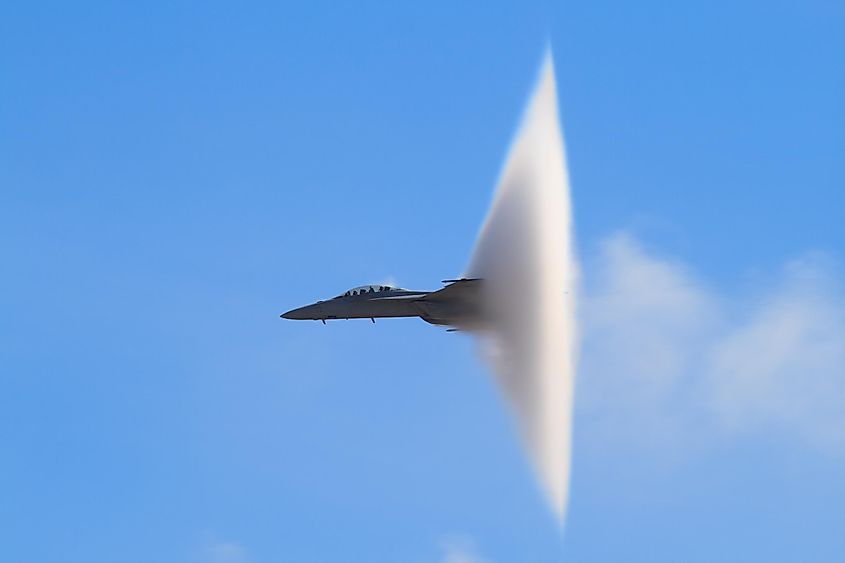
The first notable difference between the speed of sound and light is how fast they are. In Earth’s atmosphere , the speed of sound averages at about 761-miles per hour (1,225-kilometres per hour). That may seem fast, yet when compared to the speed of light, it seems quite small. Light travels at a staggering 670-million miles per hour (1.07-billion kilometres per hour). That’s around 880,000 times faster than the speed of sound.
Sound Must Have A Medium
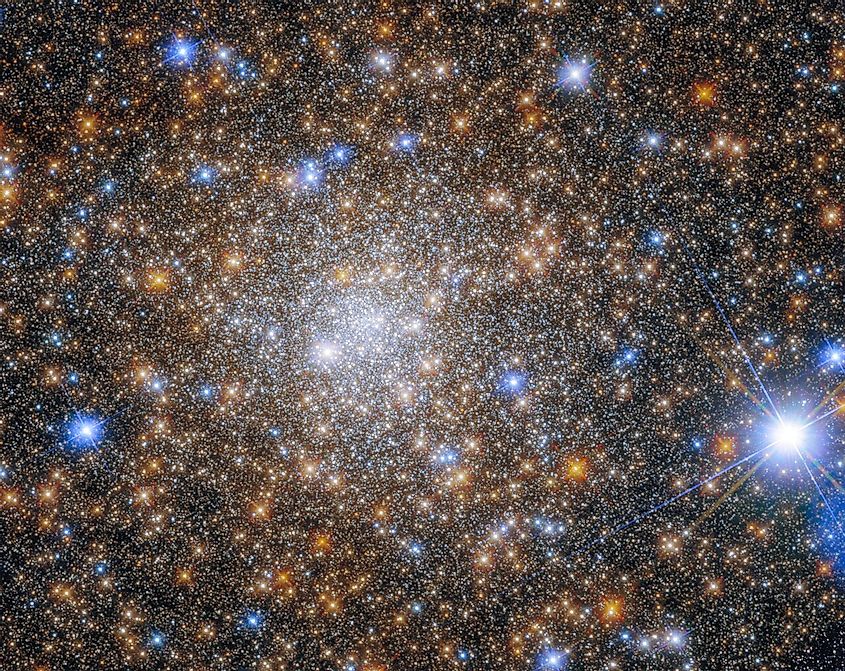
In order for sound to exist, it must have a medium to travel through. For sound, this medium is air, and that’s why sound does not exist in the emptiness of space. Meanwhile, light does not need a medium to exist. Rather, light travels independently of any medium, and thus it can travel through space, unlike sound.
The Speed Of Light Is Constant
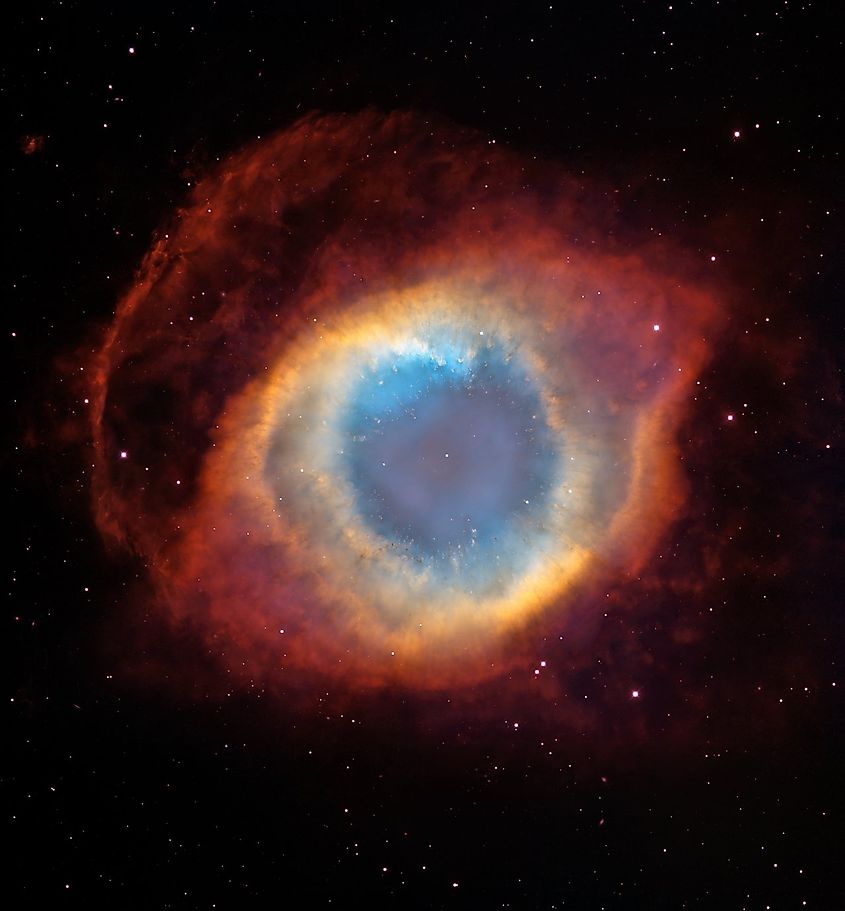
Another notable difference between light and sound is that the speed of light is constant while the speed of sound is not. Since the speed of sound requires air to exist, its speed is dependent upon the density and temperature of air. For example, when we say that sound travels at 761-miles per hour, this is only the case at sea level and when temperatures are around 59-degrees Fahrenheit (15-degrees Celsius). If you are at a higher elevation where the density of air is lower and temperatures are colder, the speed of sound will be different. Thus, the speed of sound varies. Light, however, has no such constraint. Rather, the speed of light is constant regardless of any other factor. No matter the conditions, it always travels at the same speed.
Things That Can Move Faster Than Sound
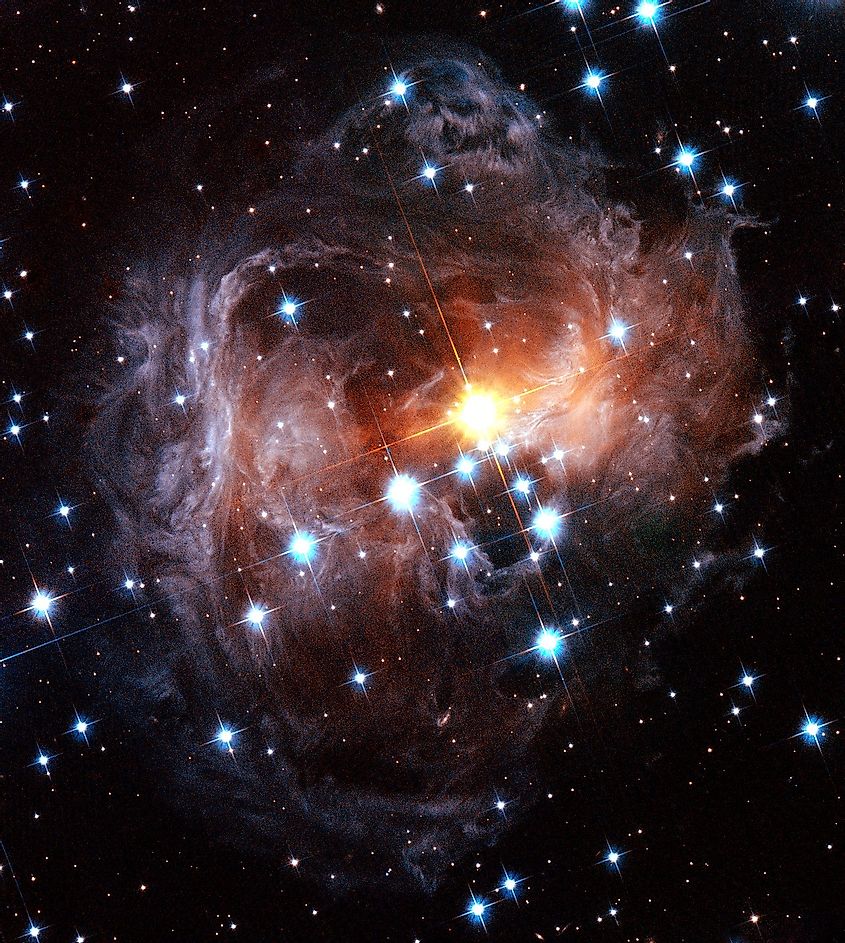
The speed of sound is fast, yet it is by no means the fastest thing in the universe. It is not even the fastest thing on Earth . Human’s have broken the sound barrier countless times, a speed known as supersonic speed. Light is a different story, however. The speed of light is a fixed law of nature, and it represents the fastest possible speed anything can move at. No matter how hard we try or how advanced technology becomes, the current understanding of the cosmos is that the light barrier cannot be broken. Thus, the speed of light is like a cosmic speed limit.
More in Science
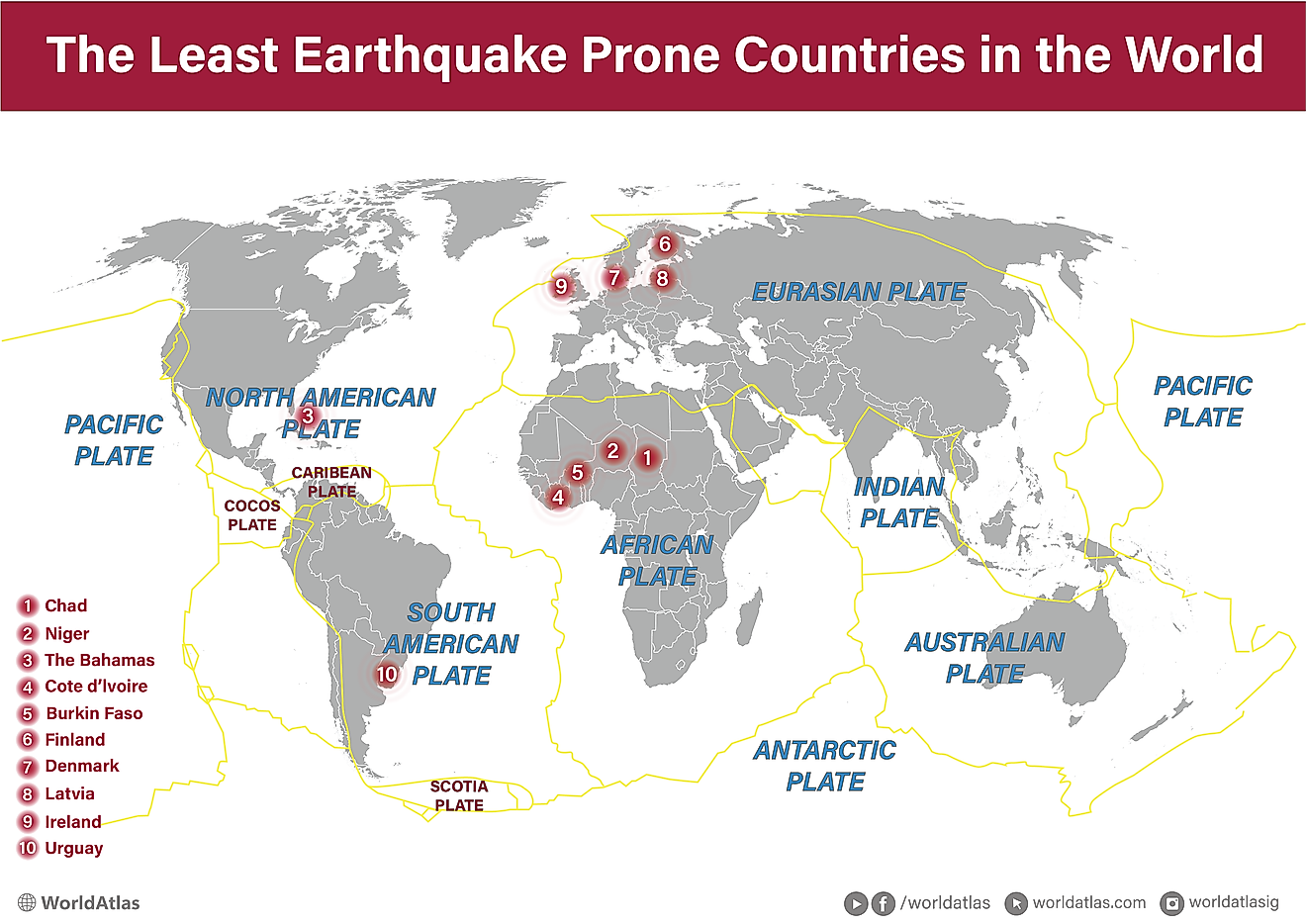
What Countries Have The Least Risk Of Earthquakes?
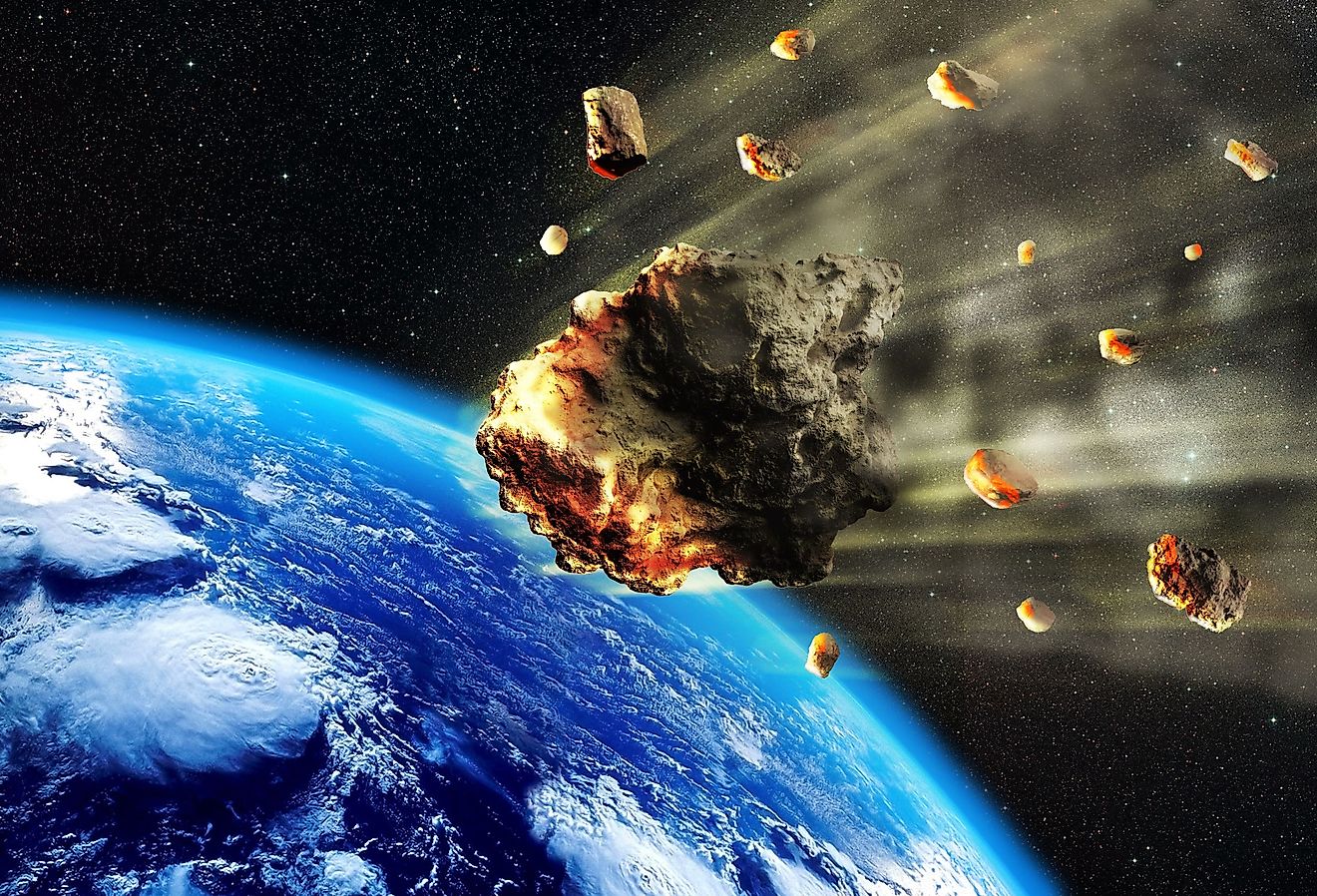
Where Do Most Earthquakes Occur In The US?
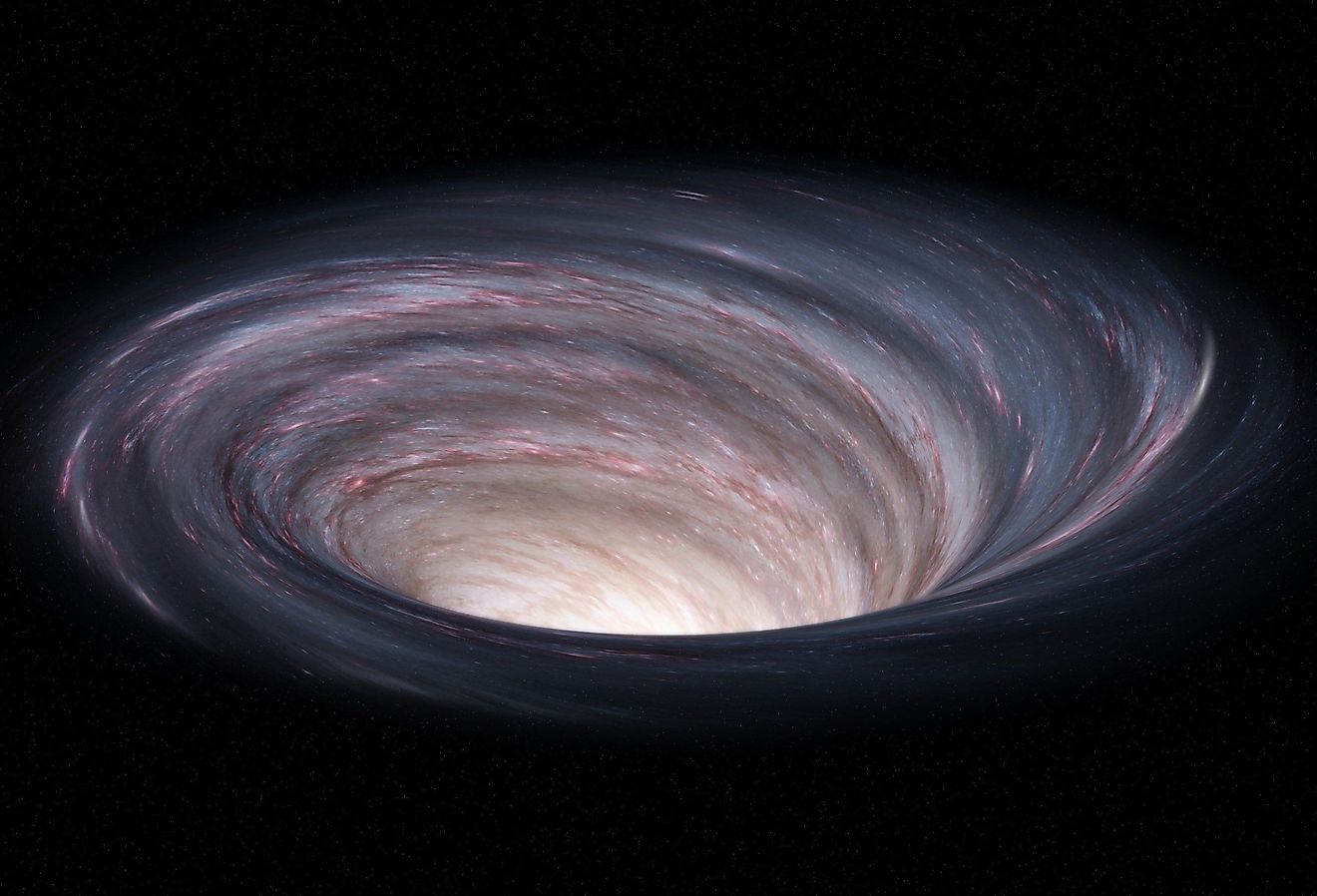
Black Holes Might be Defects in Space and Time
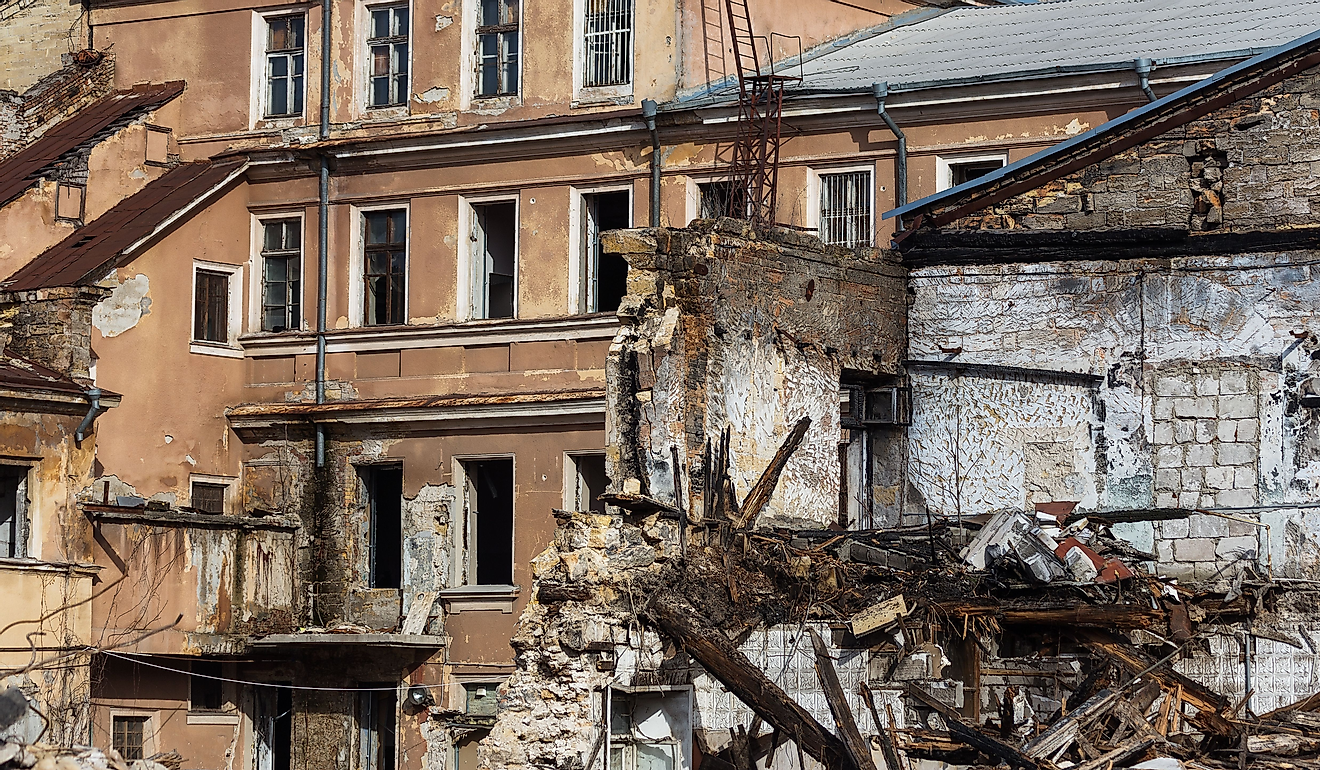
How Are Earthquakes Measured Using The Richter Scale?
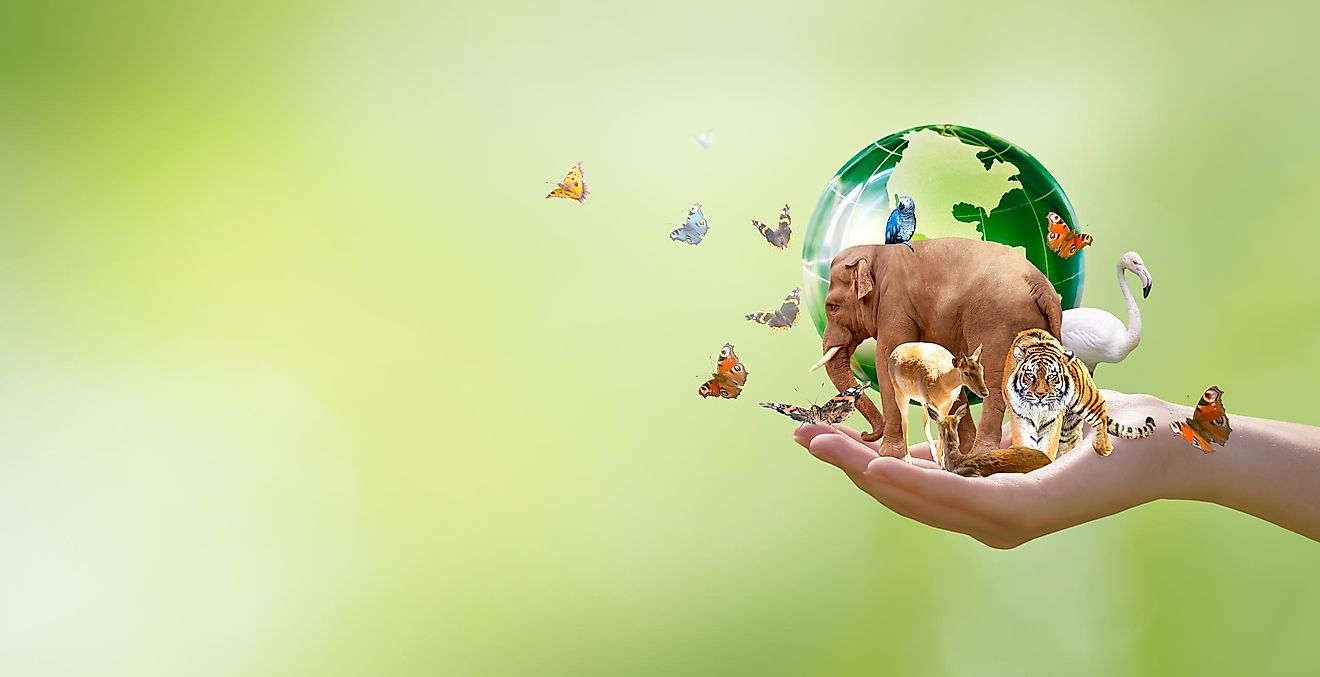
How Many New Species Are Discovered Every Year?
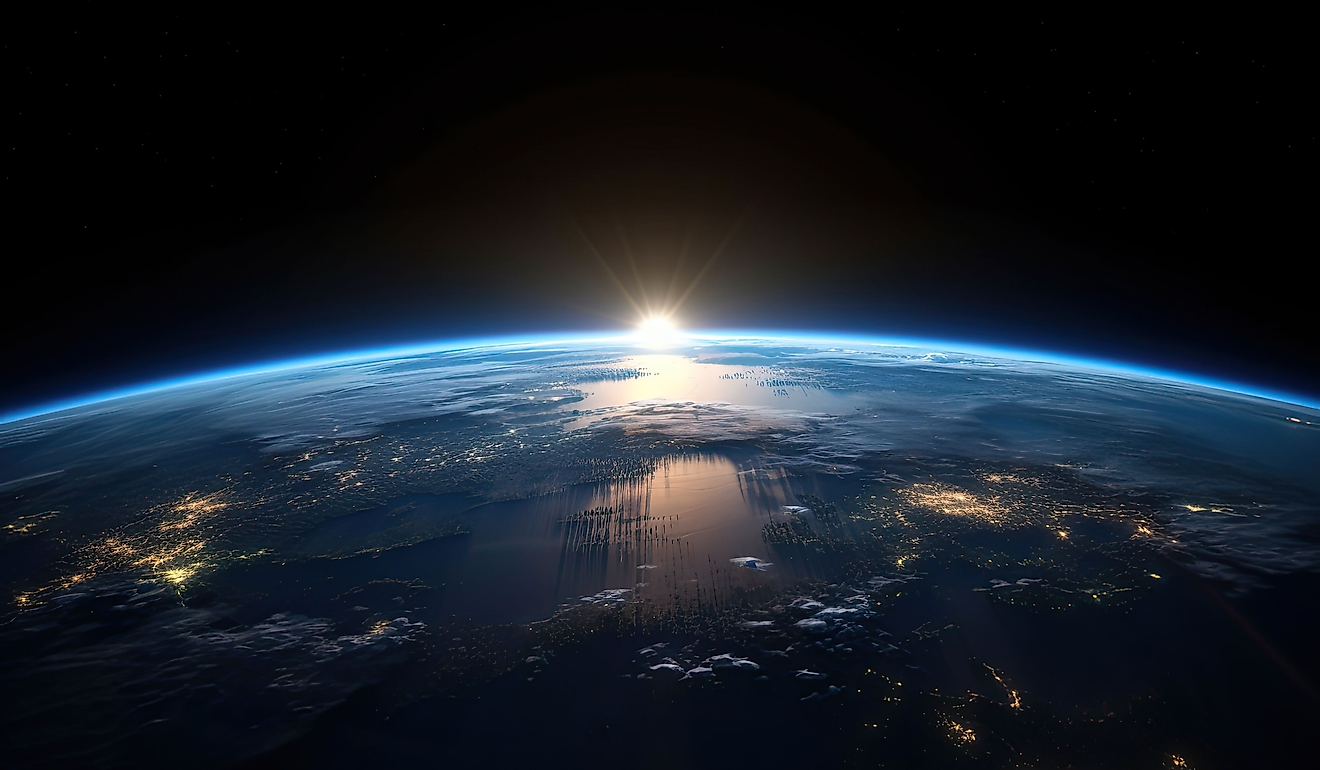
Where Does The Sun Rise And Set?
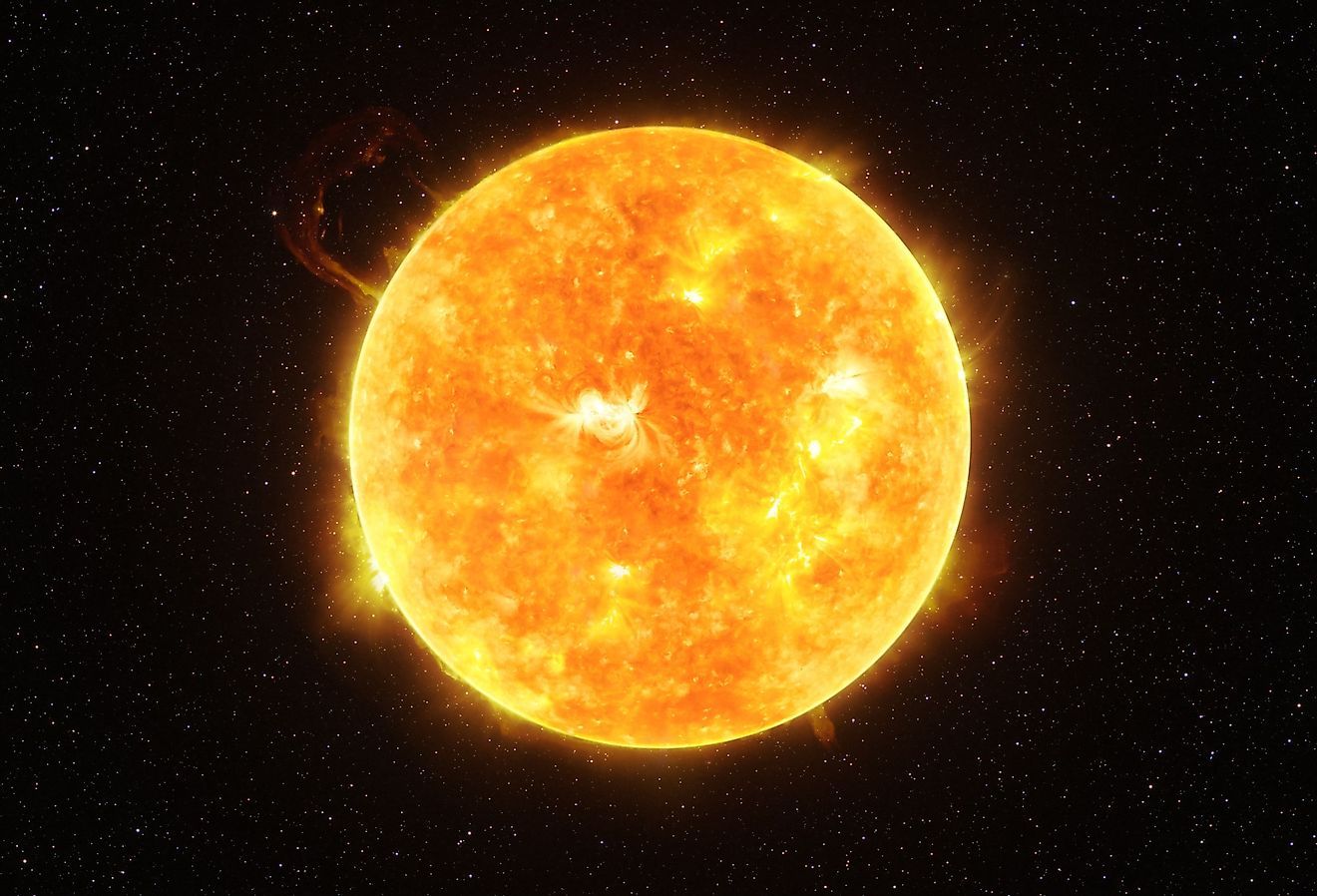
How Hot Is The Sun?
- Subscribe to BBC Science Focus Magazine
- Previous Issues
- Future tech
- Everyday science
- Planet Earth
- Newsletters
Why does light travel faster than sound?
Asked by: Toby Graham, Shrewsbury
Robert Matthews
According to Einstein's Special Relativity, the speed of light has a unique status: it's a fundamental feature of our Universe, representing the maximum speed at which information can travel from place to place. As such, nothing can match the 300,000km/s achieved by light travelling through a vacuum – least of all sound, which being waves of compression and expansion in a substance doesn’t even exist in a vacuum.
That said, light can be slowed down by being passed through transparent materials – by around 33 per cent in the case of glass. Even so, it still zooms through glass around 50,000 times faster than sound waves.
- Does the speed of light ever change?
- What would you see if you could travel at the speed of light?
- How fast does sound travel through water?
- Does sound travel further on foggy days?
Share this article

- Terms & Conditions
- Privacy policy
- Cookies policy
- Code of conduct
- Magazine subscriptions
- Manage preferences
JAVASCRIPT IS DISABLED. Please enable JavaScript on your browser to best view this site.
The Science Site
Common entrance science revision.
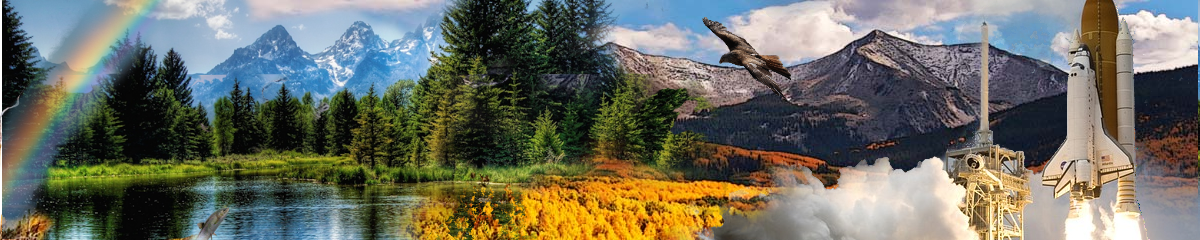
Light and Sound
- The Earth in Space
- Kinetic Theory
- Measurement
- The Bunsen Burner
- Materials and their properties
- The Sun and the Water Cycle
- Separating mixtures
- Heating chemicals
- Acids and Alkalis
- Activity series
- Elements in the Earths crust
- Gases in the Air
- Useful chemical reactions
- The Microscope
- Classification
- Life Processes
- Structure of a flowering plant
- Making Seeds
- Photosynthesis
- Blood circulation
- Breathing and respiratory system
- Food and Digestion
- Keeping Healthy
- Main organs
- Reproduction
- The carbon cycle
- micro-organisms
- Easier questions
- Average ability
- Harder questions
- 10 physics questions
- 10 chemistry questions
- 10 biology questions
- All questions – Choose your own topic
- Multiple level – timed
- Science Dictionary
- Games and simulations
- Revision tips
- Useful Links
Light and sound are made of WAVES. They are both forms of ENERGY.
Light travels much faster than sound. This is why the flash of lightening is seen long before the sound of thunder is heard, even though they are formed at the same instant.
Speed of light 3×10 10 m/s (300,000 km/s) Speed of sound 330m/s
Light Light is given out from a luminous source eg the sun or a projector lamp.
Light travels in straight lines.
In order to see anything light has to be reflected off of it and enter our eye.
Shadows are formed when RAYS of light are stopped by an object that does not TRANSMIT light.
When light hits some coloured paper (eg a red book) some colours are absorbed but the red light is scattered which is why the book looks red.
Words you need to know from this topic REFLECTION When light bounces off a smooth surface (eg a mirror) and forms an image behind it.
REFRACTION When light gets bent by passing from air into water or glass (or passing back again).
ABSORPTION When light hits an object and does not get reflected back (eg when light hits a piece of black paper it is absorbed, this is why the paper looks black)
TRANSMISSION When light passes straight through something like a piece of transparent paper.
DISPERSION The splitting of white light into a SPECTRUM. This is often done by a using a PRISM
FIBRE OPTICS: Optical fibres are strands of thin glass. Light can bounce from one end of the strand and come out of the other.
They are used in communications where they are now used to carry telephone or computer messages instead of wires.
Some ray diagrams
Sound
In order to produce a sound something has to vibrate .
The vibrating object causes compressions in the air which in turn cause the ear drum in our ear to vibrate.
The frequency of the vibrations determine the pitch of the note: Faster vibrations produce a note with a higher pitch.
The size (amplitude) of the vibrations determine the volume of the sound: If the amplitude increases then the sound will get louder .
Sound travels faster in solids and liquids than it does in air.
Sound will NOT travel through a vacuum.
How sound is produced in different musical instruments
instrument part which vibrates instrument part which vibrates
Trumpet: Lips Organ: Air
Clarinet: Reed Guitar: Strings
Piano: Strings Drum: drum skin
Echoes An echo is heard when sound is reflected off a distant object.
Sonar make use of echoes to measure the distance (or shape) of an object (eg the sea floor).
It does this by measuring the length of time it takes to hear the echo.
Ultrasound Ultra sound is too high for us to hear (maybe about 40kHz). It is used to produce pictures of unborn babies,
in burglar alarms and also in some cleaning devices
Words to know:
Frequency: the number of vibrations per second.
Pitch: how high or low a note sounds.
Amplitude: the height of a wave
Volume: How loud a note is.
If the frequency increases then the pitch will increase. If the amplitude increases then the volume will increase.
Some waveforms
14.1 Speed of Sound, Frequency, and Wavelength
Section learning objectives.
By the end of this section, you will be able to do the following:
- Relate the characteristics of waves to properties of sound waves
- Describe the speed of sound and how it changes in various media
- Relate the speed of sound to frequency and wavelength of a sound wave
Teacher Support
The learning objectives in this section will help your students master the following standards:
- (A) examine and describe oscillatory motion and wave propagation in various types of media;
- (B) investigate and analyze characteristics of waves, including velocity, frequency, amplitude, and wavelength, and calculate using the relationship between wave speed, frequency, and wavelength;
- (C) compare characteristics and behaviors of transverse waves, including electromagnetic waves and the electromagnetic spectrum, and characteristics and behaviors of longitudinal waves, including sound waves;
- (F) describe the role of wave characteristics and behaviors in medical and industrial applications.
In addition, the High School Physics Laboratory Manual addresses content in this section in the lab titled: Waves, as well as the following standards:
- (B) investigate and analyze characteristics of waves, including velocity, frequency, amplitude, and wavelength, and calculate using the relationship between wave speed, frequency, and wavelength.
Section Key Terms
[BL] [OL] Review waves and types of waves—mechanical and non-mechanical, transverse and longitudinal, pulse and periodic. Review properties of waves—amplitude, period, frequency, velocity and their inter-relations.
Properties of Sound Waves
Sound is a wave. More specifically, sound is defined to be a disturbance of matter that is transmitted from its source outward. A disturbance is anything that is moved from its state of equilibrium. Some sound waves can be characterized as periodic waves, which means that the atoms that make up the matter experience simple harmonic motion .
A vibrating string produces a sound wave as illustrated in Figure 14.2 , Figure 14.3 , and Figure 14.4 . As the string oscillates back and forth, part of the string’s energy goes into compressing and expanding the surrounding air. This creates slightly higher and lower pressures. The higher pressure... regions are compressions, and the low pressure regions are rarefactions . The pressure disturbance moves through the air as longitudinal waves with the same frequency as the string. Some of the energy is lost in the form of thermal energy transferred to the air. You may recall from the chapter on waves that areas of compression and rarefaction in longitudinal waves (such as sound) are analogous to crests and troughs in transverse waves .
The amplitude of a sound wave decreases with distance from its source, because the energy of the wave is spread over a larger and larger area. But some of the energy is also absorbed by objects, such as the eardrum in Figure 14.5 , and some of the energy is converted to thermal energy in the air. Figure 14.4 shows a graph of gauge pressure versus distance from the vibrating string. From this figure, you can see that the compression of a longitudinal wave is analogous to the peak of a transverse wave, and the rarefaction of a longitudinal wave is analogous to the trough of a transverse wave. Just as a transverse wave alternates between peaks and troughs, a longitudinal wave alternates between compression and rarefaction.
The Speed of Sound
[BL] Review the fact that sound is a mechanical wave and requires a medium through which it is transmitted.
[OL] [AL] Ask students if they know the speed of sound and if not, ask them to take a guess. Ask them why the sound of thunder is heard much after the lightning is seen during storms. This phenomenon is also observed during a display of fireworks. Through this discussion, develop the concept that the speed of sound is finite and measurable and is much slower than that of light.
The speed of sound varies greatly depending upon the medium it is traveling through. The speed of sound in a medium is determined by a combination of the medium’s rigidity (or compressibility in gases) and its density. The more rigid (or less compressible) the medium, the faster the speed of sound. The greater the density of a medium, the slower the speed of sound. The speed of sound in air is low, because air is compressible. Because liquids and solids are relatively rigid and very difficult to compress, the speed of sound in such media is generally greater than in gases. Table 14.1 shows the speed of sound in various media. Since temperature affects density, the speed of sound varies with the temperature of the medium through which it’s traveling to some extent, especially for gases.
Misconception Alert
Students might be confused between rigidity and density and how they affect the speed of sound. The speed of sound is slower in denser media. Solids are denser than gases. However, they are also very rigid, and hence sound travels faster in solids. Stress on the fact that the speed of sound always depends on a combination of these two properties of any medium.
[BL] Note that in the table, the speed of sound in very rigid materials such as glass, aluminum, and steel ... is quite high, whereas the speed in rubber, which is considerably less rigid, is quite low.
The Relationship Between the Speed of Sound and the Frequency and Wavelength of a Sound Wave
Sound, like all waves, travels at certain speeds through different media and has the properties of frequency and wavelength . Sound travels much slower than light—you can observe this while watching a fireworks display (see Figure 14.6 ), since the flash of an explosion is seen before its sound is heard.
The relationship between the speed of sound, its frequency, and wavelength is the same as for all waves:
where v is the speed of sound (in units of m/s), f is its frequency (in units of hertz), and λ λ is its wavelength (in units of meters). Recall that wavelength is defined as the distance between adjacent identical parts of a wave. The wavelength of a sound, therefore, is the distance between adjacent identical parts of a sound wave. Just as the distance between adjacent crests in a transverse wave is one wavelength, the distance between adjacent compressions in a sound wave is also one wavelength, as shown in Figure 14.7 . The frequency of a sound wave is the same as that of the source. For example, a tuning fork vibrating at a given frequency would produce sound waves that oscillate at that same frequency. The frequency of a sound is the number of waves that pass a point per unit time.
[BL] [OL] [AL] In musical instruments, shorter strings vibrate faster and hence produce sounds at higher pitches. Fret placements on instruments such as guitars, banjos, and mandolins, are mathematically determined to give the correct interval or change in pitch. When the string is pushed against the fret wire, the string is effectively shortened, changing its pitch. Ask students to experiment with strings of different lengths and observe how the pitch changes in each case.
One of the more important properties of sound is that its speed is nearly independent of frequency. If this were not the case, and high-frequency sounds traveled faster, for example, then the farther you were from a band in a football stadium, the more the sound from the low-pitch instruments would lag behind the high-pitch ones. But the music from all instruments arrives in cadence independent of distance, and so all frequencies must travel at nearly the same speed.
Recall that v = f λ v = f λ , and in a given medium under fixed temperature and humidity, v is constant. Therefore, the relationship between f and λ λ is inverse: The higher the frequency, the shorter the wavelength of a sound wave.
Teacher Demonstration
Hold a meter stick flat on a desktop, with about 80 cm sticking out over the edge of the desk. Make the meter stick vibrate by pulling the tip down and releasing, while holding the meter stick tight to the desktop. While it is vibrating, move the stick back onto the desktop, shortening the part that is sticking out. Students will see the shortening of the vibrating part of the meter stick, and hear the pitch or number of vibrations go up—an increase in frequency.
The speed of sound can change when sound travels from one medium to another. However, the frequency usually remains the same because it is like a driven oscillation and maintains the frequency of the original source. If v changes and f remains the same, then the wavelength λ λ must change. Since v = f λ v = f λ , the higher the speed of a sound, the greater its wavelength for a given frequency.
[AL] Ask students to predict what would happen if the speeds of sound in air varied by frequency.
Virtual Physics
This simulation lets you see sound waves. Adjust the frequency or amplitude (volume) and you can see and hear how the wave changes. Move the listener around and hear what she hears. Switch to the Two Source Interference tab or the Interference by Reflection tab to experiment with interference and reflection.
Tips For Success
Make sure to have audio enabled and set to Listener rather than Speaker, or else the sound will not vary as you move the listener around.
- Because, intensity of the sound wave changes with the frequency.
- Because, the speed of the sound wave changes when the frequency is changed.
- Because, loudness of the sound wave takes time to adjust after a change in frequency.
- Because it takes time for sound to reach the listener, so the listener perceives the new frequency of sound wave after a delay.
- Yes, the speed of propagation depends only on the frequency of the wave.
- Yes, the speed of propagation depends upon the wavelength of the wave, and wavelength changes as the frequency changes.
- No, the speed of propagation depends only on the wavelength of the wave.
- No, the speed of propagation is constant in a given medium; only the wavelength changes as the frequency changes.
Voice as a Sound Wave
In this lab you will observe the effects of blowing and speaking into a piece of paper in order to compare and contrast different sound waves.
- sheet of paper
Instructions
- Suspend a sheet of paper so that the top edge of the paper is fixed and the bottom edge is free to move. You could tape the top edge of the paper to the edge of a table, for example.
- Gently blow air near the edge of the bottom of the sheet and note how the sheet moves.
- Speak softly and then louder such that the sounds hit the edge of the bottom of the paper, and note how the sheet moves.
- Interpret the results.
Grasp Check
Which sound wave property increases when you are speaking more loudly than softly?
- amplitude of the wave
- frequency of the wave
- speed of the wave
- wavelength of the wave
Worked Example
What are the wavelengths of audible sounds.
Calculate the wavelengths of sounds at the extremes of the audible range, 20 and 20,000 Hz, in conditions where sound travels at 348.7 m/s.
To find wavelength from frequency, we can use v = f λ v = f λ .
(1) Identify the knowns. The values for v and f are given.
(2) Solve the relationship between speed, frequency and wavelength for λ λ .
(3) Enter the speed and the minimum frequency to give the maximum wavelength.
(4) Enter the speed and the maximum frequency to give the minimum wavelength.
Because the product of f multiplied by λ λ equals a constant velocity in unchanging conditions, the smaller f is, the larger λ λ must be, and vice versa. Note that you can also easily rearrange the same formula to find frequency or velocity.
Practice Problems
- 5 × 10 3 m / s
- 3.2 × 10 2 m / s
- 2 × 10 − 4 m/s
- 8 × 10 2 m / s
- 2.0 × 10 7 m
- 1.5 × 10 7 m
- 1.4 × 10 2 m
- 7.4 × 10 − 3 m
Links To Physics
Echolocation.
Echolocation is the use of reflected sound waves to locate and identify objects. It is used by animals such as bats, dolphins and whales, and is also imitated by humans in SONAR—Sound Navigation and Ranging—and echolocation technology.
Bats, dolphins and whales use echolocation to navigate and find food in their environment. They locate an object (or obstacle) by emitting a sound and then sensing the reflected sound waves. Since the speed of sound in air is constant, the time it takes for the sound to travel to the object and back gives the animal a sense of the distance between itself and the object. This is called ranging . Figure 14.8 shows a bat using echolocation to sense distances.
Echolocating animals identify an object by comparing the relative intensity of the sound waves returning to each ear to figure out the angle at which the sound waves were reflected. This gives information about the direction, size and shape of the object. Since there is a slight distance in position between the two ears of an animal, the sound may return to one of the ears with a bit of a delay, which also provides information about the position of the object. For example, if a bear is directly to the right of a bat, the echo will return to the bat’s left ear later than to its right ear. If, however, the bear is directly ahead of the bat, the echo would return to both ears at the same time. For an animal without a sense of sight such as a bat, it is important to know where other animals are as well as what they are; their survival depends on it.
Principles of echolocation have been used to develop a variety of useful sensing technologies. SONAR, is used by submarines to detect objects underwater and measure water depth. Unlike animal echolocation, which relies on only one transmitter (a mouth) and two receivers (ears), manmade SONAR uses many transmitters and beams to get a more accurate reading of the environment. Radar technologies use the echo of radio waves to locate clouds and storm systems in weather forecasting, and to locate aircraft for air traffic control. Some new cars use echolocation technology to sense obstacles around the car, and warn the driver who may be about to hit something (or even to automatically parallel park). Echolocation technologies and training systems are being developed to help visually impaired people navigate their everyday environments.
- The echo would return to the left ear first.
- The echo would return to the right ear first.
Check Your Understanding
Use these questions to assess student achievement of the section’s Learning Objectives. If students are struggling with a specific objective, these questions will help identify which and direct students to the relevant content.
- Rarefaction is the high-pressure region created in a medium when a longitudinal wave passes through it.
- Rarefaction is the low-pressure region created in a medium when a longitudinal wave passes through it.
- Rarefaction is the highest point of amplitude of a sound wave.
- Rarefaction is the lowest point of amplitude of a sound wave.
What sort of motion do the particles of a medium experience when a sound wave passes through it?
- Simple harmonic motion
- Circular motion
- Random motion
- Translational motion
What does the speed of sound depend on?
- The wavelength of the wave
- The size of the medium
- The frequency of the wave
- The properties of the medium
What property of a gas would affect the speed of sound traveling through it?
- The volume of the gas
- The flammability of the gas
- The mass of the gas
- The compressibility of the gas
As an Amazon Associate we earn from qualifying purchases.
This book may not be used in the training of large language models or otherwise be ingested into large language models or generative AI offerings without OpenStax's permission.
Want to cite, share, or modify this book? This book uses the Creative Commons Attribution License and you must attribute Texas Education Agency (TEA). The original material is available at: https://www.texasgateway.org/book/tea-physics . Changes were made to the original material, including updates to art, structure, and other content updates.
Access for free at https://openstax.org/books/physics/pages/1-introduction
- Authors: Paul Peter Urone, Roger Hinrichs
- Publisher/website: OpenStax
- Book title: Physics
- Publication date: Mar 26, 2020
- Location: Houston, Texas
- Book URL: https://openstax.org/books/physics/pages/1-introduction
- Section URL: https://openstax.org/books/physics/pages/14-1-speed-of-sound-frequency-and-wavelength
© Jan 19, 2024 Texas Education Agency (TEA). The OpenStax name, OpenStax logo, OpenStax book covers, OpenStax CNX name, and OpenStax CNX logo are not subject to the Creative Commons license and may not be reproduced without the prior and express written consent of Rice University.
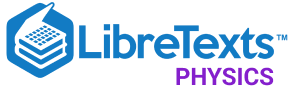
- school Campus Bookshelves
- menu_book Bookshelves
- perm_media Learning Objects
- login Login
- how_to_reg Request Instructor Account
- hub Instructor Commons
- Download Page (PDF)
- Download Full Book (PDF)
- Periodic Table
- Physics Constants
- Scientific Calculator
- Reference & Cite
- Tools expand_more
- Readability
selected template will load here
This action is not available.
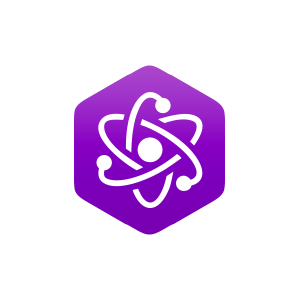
17.2: Speed of Sound, Frequency, and Wavelength
- Last updated
- Save as PDF
- Page ID 1615
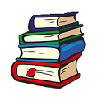
Learning Objectives
By the end of this section, you will be able to:
- Define pitch.
- Describe the relationship between the speed of sound, its frequency, and its wavelength.
- Describe the effects on the speed of sound as it travels through various media.
- Describe the effects of temperature on the speed of sound.
Sound, like all waves, travels at a certain speed and has the properties of frequency and wavelength. You can observe direct evidence of the speed of sound while watching a fireworks display. The flash of an explosion is seen well before its sound is heard, implying both that sound travels at a finite speed and that it is much slower than light. You can also directly sense the frequency of a sound. Perception of frequency is called pitch . The wavelength of sound is not directly sensed, but indirect evidence is found in the correlation of the size of musical instruments with their pitch. Small instruments, such as a piccolo, typically make high-pitch sounds, while large instruments, such as a tuba, typically make low-pitch sounds. High pitch means small wavelength, and the size of a musical instrument is directly related to the wavelengths of sound it produces. So a small instrument creates short-wavelength sounds. Similar arguments hold that a large instrument creates long-wavelength sounds.
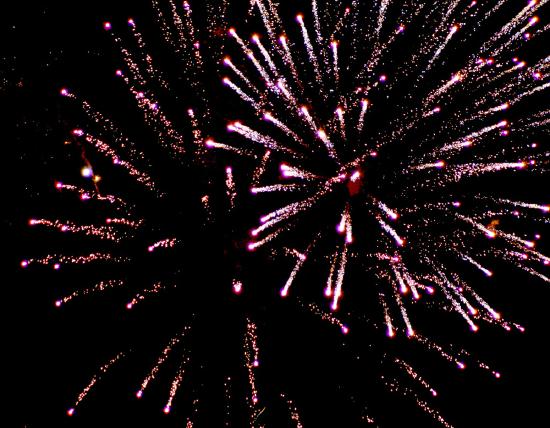
The relationship of the speed of sound, its frequency, and wavelength is the same as for all waves:
\[v_w = f\lambda,\]
where \(v_w\) is the speed of sound, \(f\) is its frequency, and \(\lambda\) is its wavelength. The wavelength of a sound is the distance between adjacent identical parts of a wave—for example, between adjacent compressions as illustrated in Figure \(\PageIndex{2}\). The frequency is the same as that of the source and is the number of waves that pass a point per unit time.
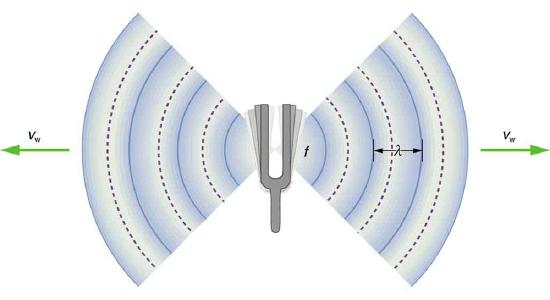
Table \(\PageIndex{1}\) makes it apparent that the speed of sound varies greatly in different media. The speed of sound in a medium is determined by a combination of the medium’s rigidity (or compressibility in gases) and its density. The more rigid (or less compressible) the medium, the faster the speed of sound. For materials that have similar rigidities, sound will travel faster through the one with the lower density because the sound energy is more easily transferred from particle to particle. The speed of sound in air is low, because air is compressible. Because liquids and solids are relatively rigid and very difficult to compress, the speed of sound in such media is generally greater than in gases.
Earthquakes, essentially sound waves in Earth’s crust, are an interesting example of how the speed of sound depends on the rigidity of the medium. Earthquakes have both longitudinal and transverse components, and these travel at different speeds. The bulk modulus of granite is greater than its shear modulus. For that reason, the speed of longitudinal or pressure waves (P-waves) in earthquakes in granite is significantly higher than the speed of transverse or shear waves (S-waves). Both components of earthquakes travel slower in less rigid material, such as sediments. P-waves have speeds of 4 to 7 km/s, and S-waves correspondingly range in speed from 2 to 5 km/s, both being faster in more rigid material. The P-wave gets progressively farther ahead of the S-wave as they travel through Earth’s crust. The time between the P- and S-waves is routinely used to determine the distance to their source, the epicenter of the earthquake.
The speed of sound is affected by temperature in a given medium. For air at sea level, the speed of sound is given by
\[v_w = (331 \, m/s)\sqrt{\dfrac{T}{273 \, K}},\]
where the temperature (denoted as \(T\)) is in units of kelvin. The speed of sound in gases is related to the average speed of particles in the gas, \(v_{rms}\), and that
\[v_{rms} = \sqrt{\dfrac{3 \, kT}{m}},\]
where \(k\) is the Boltzmann constant \((1.38 \times 10^{-23} \, J/K)\) and \(m\) is the mass of each (identical) particle in the gas. So, it is reasonable that the speed of sound in air and other gases should depend on the square root of temperature. While not negligible, this is not a strong dependence. At \(0^oC\), the speed of sound is 331 m/s, whereas at \(20^oC\) it is 343 m/s, less than a 4% increase. Figure \(\PageIndex{3}\) shows a use of the speed of sound by a bat to sense distances. Echoes are also used in medical imaging.
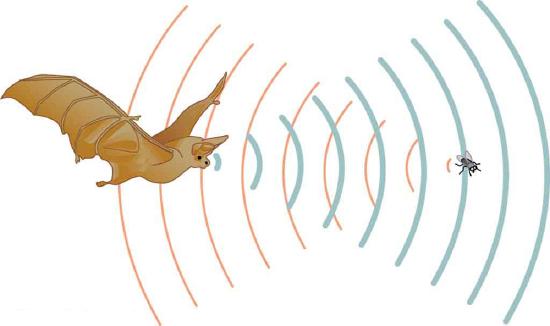
One of the more important properties of sound is that its speed is nearly independent of frequency. This independence is certainly true in open air for sounds in the audible range of 20 to 20,000 Hz. If this independence were not true, you would certainly notice it for music played by a marching band in a football stadium, for example. Suppose that high-frequency sounds traveled faster—then the farther you were from the band, the more the sound from the low-pitch instruments would lag that from the high-pitch ones. But the music from all instruments arrives in cadence independent of distance, and so all frequencies must travel at nearly the same speed. Recall that
\[v_w = f\lambda.\]
In a given medium under fixed conditions, \(v_w\) is constant, so that there is a relationship between \(f\) and \(\lambda\); the higher the frequency, the smaller the wavelength. See Figure \(\PageIndex{4}\) and consider the following example.
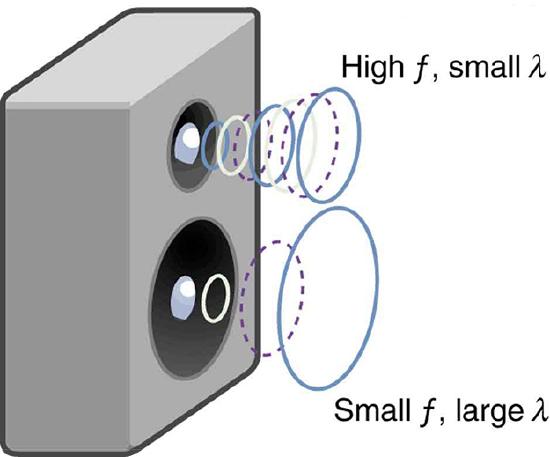
Example \(\PageIndex{1}\): Calculating Wavelengths: What Are the Wavelengths of Audible Sounds?
Calculate the wavelengths of sounds at the extremes of the audible range, 20 and 20,000 Hz, in \(30.0^oC\) air. (Assume that the frequency values are accurate to two significant figures.)
To find wavelength from frequency, we can use \(v_w = f\lambda\).
- Identify knowns. The value for \(v_w\), is given by \[v_w = (331 \, m/s)\sqrt{\dfrac{T}{273 \, K}}. \nonumber\]
- Convert the temperature into kelvin and then enter the temperature into the equation \[v_w = (331 \, m/s)\sqrt{\dfrac{303 \, K}{273 \, K}} = 348.7 \, m/s. \nonumber\]
- Solve the relationship between speed and wavelength for \(\lambda\): \[\lambda = \dfrac{v_w}{f}. \nonumber \]
- Enter the speed and the minimum frequency to give the maximum wavelength: \[\lambda_{max} = \dfrac{348.7 \, m/s}{20 \, Hz} = 17 \, m. \nonumber\]
- Enter the speed and the maximum frequency to give the minimum wavelength: \[\lambda_{min} = \dfrac{348.7 \, m/s}{20,000 \, Hz} = 0.017 \, m = 1.7 \, cm. \nonumber\]
Because the product of \(f\) multiplied by \(\lambda\) equals a constant, the smaller \(f\) is, the larger \(\lambda\) must be, and vice versa.
The speed of sound can change when sound travels from one medium to another. However, the frequency usually remains the same because it is like a driven oscillation and has the frequency of the original source. If \(v_w\) changes and \(f\) remains the same, then the wavelength \(\lambda\) must change. That is, because \(v_w = f\lambda\), the higher the speed of a sound, the greater its wavelength for a given frequency.
MAKING CONNECTIONS: TAKE-HOME INVESTIGATION - VOICE AS A SOUND WAVE
Suspend a sheet of paper so that the top edge of the paper is fixed and the bottom edge is free to move. You could tape the top edge of the paper to the edge of a table. Gently blow near the edge of the bottom of the sheet and note how the sheet moves. Speak softly and then louder such that the sounds hit the edge of the bottom of the paper, and note how the sheet moves. Explain the effects.
Exercise \(\PageIndex{1A}\)
Imagine you observe two fireworks explode. You hear the explosion of one as soon as you see it. However, you see the other firework for several milliseconds before you hear the explosion. Explain why this is so.
Sound and light both travel at definite speeds. The speed of sound is slower than the speed of light. The first firework is probably very close by, so the speed difference is not noticeable. The second firework is farther away, so the light arrives at your eyes noticeably sooner than the sound wave arrives at your ears.
Exercise \(\PageIndex{1B}\)
You observe two musical instruments that you cannot identify. One plays high-pitch sounds and the other plays low-pitch sounds. How could you determine which is which without hearing either of them play?
Compare their sizes. High-pitch instruments are generally smaller than low-pitch instruments because they generate a smaller wavelength.
- The relationship of the speed of sound \(v_w\), its frequency \(f\), and its wavelength \(\lambda\) is given by \(v_w = f\lambda,\) which is the same relationship given for all waves.
- In air, the speed of sound is related to air temperature \(T\) by \(v_w = (331 \, m/s) \sqrt{\dfrac{T}{273 \, K}}.\) \(v_w\) is the same for all frequencies and wavelengths.
Blue Sky Science: Why is light faster than sound?
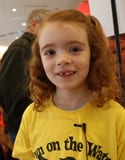
Molly Torinus
Why is light faster than sound?
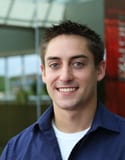
Brandon Walker
Light and sound are very different. Sound is actually a mechanical disturbance through air or another medium. Sound always needs a medium to travel through and the type of medium determines its speed.
Imagine a bunch of molecules bouncing around in the air. If you hit an object or make a fast motion, the molecules that you push are going to hit the ones in front of it. You’ll get this disturbance in the direction of travel of however you made the initial motion, and it will move through the medium. That’s how sound travels—as a pressure wave.
Light, on the other hand, is not a pressure wave—it’s a fundamental particle. One ray of light is typically called a photon, and it’s an electromagnetic disturbance. Light doesn’t need a medium to travel.
The speed of sound through air is about 340 meters per second. It’s faster through water and it’s even faster through steel. Light will travel through a vacuum at 300 million meters per second. So they’re totally different scales.
No information can propagate faster than the speed of light. If you have light that’s going through a media, it can travel slower than that. But the speed of sound and speed of light are totally incomparable.
You normally don’t notice this speed difference on a day-to-day basis. This speed difference does become apparent, for example, with lightning. You’ll always see lightning before you hear it, because typically lightning will be a mile away, two miles away. That’s a great enough distance that that speed difference becomes apparent to your brain.
NOTIFICATIONS
Light basics.
- + Create new collection
Light is a form of energy produced by a light source. Light is made of photons that travel very fast. Photons of light behave like both waves and particles.
Light sources
Something that produces light is called a light source. There are two main kinds of light sources:
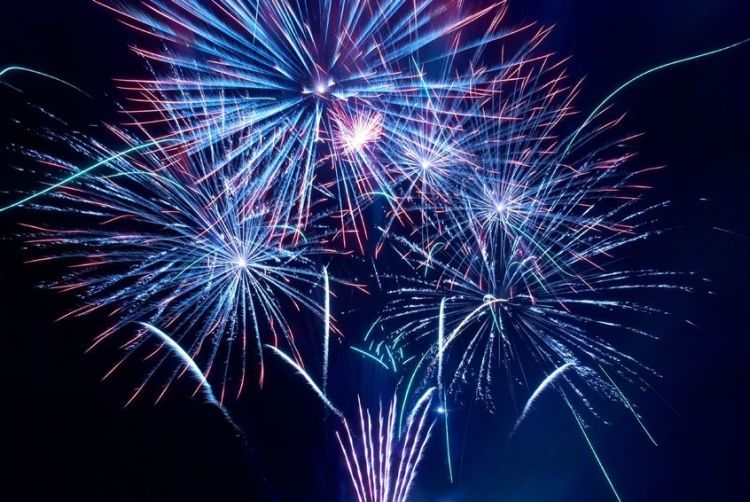
Fireworks show how light travels faster than sound. We see the light almost instantly, but the sound arrives later. To work out how many kilometres away the fireworks are, count the seconds until you hear the bang and divide by 3.
Incandescent sources use heat to produce light. Nearly all solids, liquids and gases will start to glow with a dull red colour once they reach a temperature of about 525 °C. At about 2300 °C, the filament in a light bulb will start to produce all of the colours of the visible spectrum, so it will look white. The Sun, stars, a flame and molten metal are all incandescent.
Luminescent sources are normally cooler and can be produced by chemical reactions, such as in a glowstick or a glow-worm. Other luminescent sources include a computer screen, fluorescent lights and LEDs.
Light travels much faster than sound
Light travels at a speed of 299,792,458 m/s (that’s nearly 300,000 km/s!). The distance around the Earth is 40,000 km, so in 1 second, light could travel seven and a half times around the world.
Sound only travels at about 330 m/s through the air, so light is nearly a million times faster than sound.
If lightning flashes 1 kilometre away from you, the light reaches you in 3 millionths of a second, which is almost instantly. The sound of the thunder takes 3 seconds to travel 1 kilometre – to work out many kilometres away lightning is, count the seconds for the thunder to arrive and divide by 3.
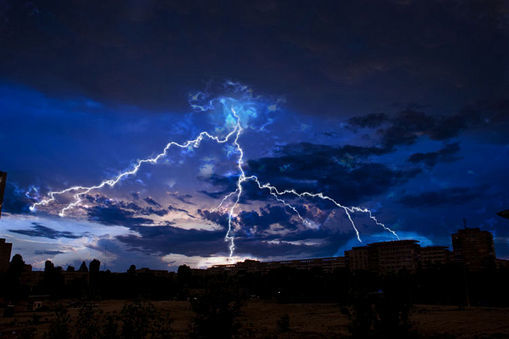
Lightning storms are important for converting nitrogen gas in the atmosphere through to forms that are biologically available.
Light takes about 8 minutes and 20 seconds to reach the Earth from the Sun. When we see the Sun, we are seeing what it looked like over 8 minutes ago.
Light can travel through empty space
Unlike sound, which needs a medium (like air or water) to travel through, light can travel in the vacuum of space.
Light travels in straight lines
Once light has been produced, it will keep travelling in a straight line until it hits something else.
Shadows are evidence of light travelling in straight lines. An object blocks light so that it can’t reach the surface where we see the shadow. Light fills up all of the space before it hits the object, but the whole region between the object and the surface is in shadow. Shadows don’t appear totally dark because there is still some light reaching the surface that has been reflected off other objects.
Once light has hit another surface or particles, it is then absorbed, reflected (bounces off), scattered (bounces off in all directions), refracted (direction and speed changes) or transmitted (passes straight through).
Models for light
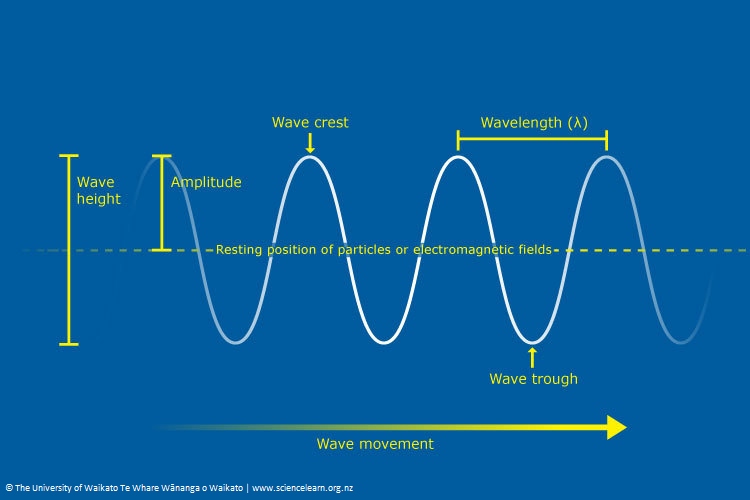
Wave length, height and frequency
A wave can be described by its length, height (amplitude) and frequency.
Light as waves
Rainbows and prisms can split white light up into different colours. Experiments can be used to show that each of these colours has a different wavelength.
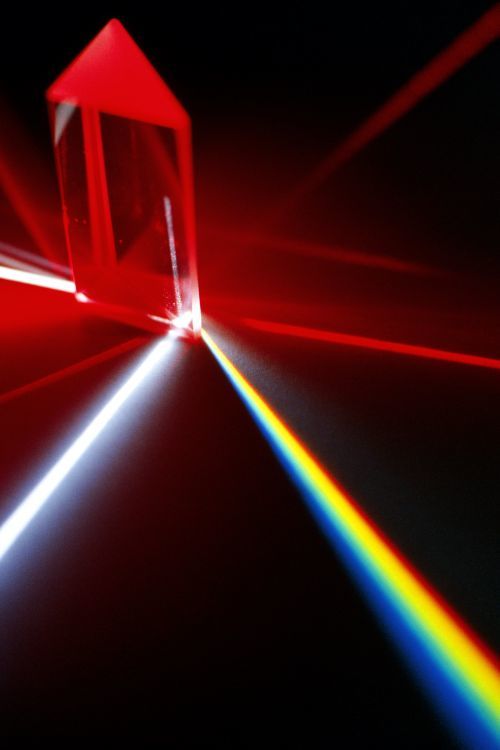
When white light shines through a prism, each colour refracts at a slightly different angle. Violet light refracts slightly more than red light. A prism can be used to show the seven colours of the spectrum that make up white light.
At the beach, the wavelength of water waves might be measured in metres, but the wavelength of light is measured in nanometres – 10 -9 (0.000,000,001) of a metre. Red light has a wavelength of nearly 700 nm (that’s 7 ten-thousandths of a millimetre) while violet light is only 400 nm (4 ten-thousandths of a millimetre).
Visible light is only a very small part of the electromagnetic spectrum – it’s just that this is the range of wavelengths our eyes can detect.
Light as particles
In 1905, Albert Einstein proposed that light is made of billions of small packets of energy that we now call photons. These photons have no mass, but each photon has a specific amount of energy that depends on its frequency (number of vibrations per second). Each photon still has a wavelength. Shorter wavelength photons have more energy.
The photoelectric effect
University of Waikato science researcher Dr Adrian Dorrington explains the photoelectric effect. He then describes how camera sensors can be designed on the basis of this effect to enable light energy to be converted into electric potential energy.
The photoelectric effect is when light can cause electrons to jump out of a metal. These experiments confirm that light is made of these massless particles called photons.
Simple explanations of some of these concepts can be found in the article Building Science Concepts: Shadows .
Nature of science
In order to understand the world we live in, scientists often use models. Sometimes, several models are needed to explain the properties and behaviours of a phenomenon. For example, to understand the behaviour of light, two models are needed. Light needs to be thought of as both waves and particles.
Useful links
Even though light doesn’t have mass, learn how it still has a tiny amount of momentum. Find out about NASA’s solar sails to power spacecraft.
Read about the LightSail project, a crowdfunded project from The Planetary Society, aiming to demonstrate that solar sailing is a viable means of propulsion for CubeSats (miniature satellites intended for low Earth orbit).
Explore solar sails more in your classroom, with this activity Solar Sails: The Future of Space Travel from the TeachEngineering website.
See our newsletters here .
Would you like to take a short survey?
This survey will open in a new tab and you can fill it out after your visit to the site.
What is the speed of light?
The speed of light is the speed limit of the universe. Or is it?
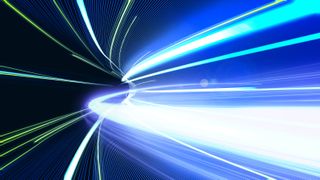
What is a light-year?
- Speed of light FAQs
- Special relativity
- Faster than light
- Slowing down light
- Faster-than-light travel
Bibliography
The speed of light traveling through a vacuum is exactly 299,792,458 meters (983,571,056 feet) per second. That's about 186,282 miles per second — a universal constant known in equations as "c," or light speed.
According to physicist Albert Einstein 's theory of special relativity , on which much of modern physics is based, nothing in the universe can travel faster than light. The theory states that as matter approaches the speed of light, the matter's mass becomes infinite. That means the speed of light functions as a speed limit on the whole universe . The speed of light is so immutable that, according to the U.S. National Institute of Standards and Technology , it is used to define international standard measurements like the meter (and by extension, the mile, the foot and the inch). Through some crafty equations, it also helps define the kilogram and the temperature unit Kelvin .
But despite the speed of light's reputation as a universal constant, scientists and science fiction writers alike spend time contemplating faster-than-light travel. So far no one's been able to demonstrate a real warp drive, but that hasn't slowed our collective hurtle toward new stories, new inventions and new realms of physics.
Related: Special relativity holds up to a high-energy test
A l ight-year is the distance that light can travel in one year — about 6 trillion miles (10 trillion kilometers). It's one way that astronomers and physicists measure immense distances across our universe.
Light travels from the moon to our eyes in about 1 second, which means the moon is about 1 light-second away. Sunlight takes about 8 minutes to reach our eyes, so the sun is about 8 light minutes away. Light from Alpha Centauri , which is the nearest star system to our own, requires roughly 4.3 years to get here, so Alpha Centauri is 4.3 light-years away.
"To obtain an idea of the size of a light-year, take the circumference of the Earth (24,900 miles), lay it out in a straight line, multiply the length of the line by 7.5 (the corresponding distance is one light-second), then place 31.6 million similar lines end to end," NASA's Glenn Research Center says on its website . "The resulting distance is almost 6 trillion (6,000,000,000,000) miles!"
Stars and other objects beyond our solar system lie anywhere from a few light-years to a few billion light-years away. And everything astronomers "see" in the distant universe is literally history. When astronomers study objects that are far away, they are seeing light that shows the objects as they existed at the time that light left them.
This principle allows astronomers to see the universe as it looked after the Big Bang , which took place about 13.8 billion years ago. Objects that are 10 billion light-years away from us appear to astronomers as they looked 10 billion years ago — relatively soon after the beginning of the universe — rather than how they appear today.
Related: Why the universe is all history
Speed of light FAQs answered by an expert
We asked Rob Zellem, exoplanet-hunter and staff scientist at NASA's Jet Propulsion Lab, a few frequently asked questions about the speed of light.
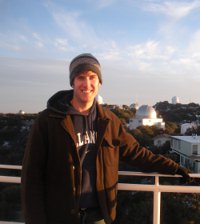
Dr. Rob Zellem is a staff scientist at NASA's Jet Propulsion Laboratory, a federally funded research and development center operated by the California Institute of Technology. Rob is the project lead for Exoplanet Watch, a citizen science project to observe exoplanets, planets outside of our own solar system, with small telescopes. He is also the Science Calibration lead for the Nancy Grace Roman Space Telescope's Coronagraph Instrument, which will directly image exoplanets.
What is faster than the speed of light?
Nothing! Light is a "universal speed limit" and, according to Einstein's theory of relativity, is the fastest speed in the universe: 300,000 kilometers per second (186,000 miles per second).
Is the speed of light constant?
The speed of light is a universal constant in a vacuum, like the vacuum of space. However, light *can* slow down slightly when it passes through an absorbing medium, like water (225,000 kilometers per second = 140,000 miles per second) or glass (200,000 kilometers per second = 124,000 miles per second).
Who discovered the speed of light?
One of the first measurements of the speed of light was by Rømer in 1676 by observing the moons of Jupiter . The speed of light was first measured to high precision in 1879 by the Michelson-Morley Experiment.
How do we know the speed of light?
Rømer was able to measure the speed of light by observing eclipses of Jupiter's moon Io. When Jupiter was closer to Earth, Rømer noted that eclipses of Io occurred slightly earlier than when Jupiter was farther away. Rømer attributed this effect due the time it takes for light to travel over the longer distance when Jupiter was farther from the Earth.
How did we learn the speed of light?
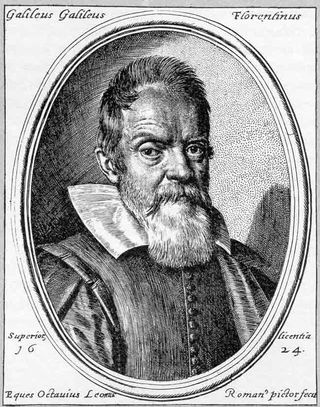
As early as the 5th century BC, Greek philosophers like Empedocles and Aristotle disagreed on the nature of light speed. Empedocles proposed that light, whatever it was made of, must travel and therefore, must have a rate of travel. Aristotle wrote a rebuttal of Empedocles' view in his own treatise, On Sense and the Sensible , arguing that light, unlike sound and smell, must be instantaneous. Aristotle was wrong, of course, but it would take hundreds of years for anyone to prove it.
In the mid 1600s, the Italian astronomer Galileo Galilei stood two people on hills less than a mile apart. Each person held a shielded lantern. One uncovered his lantern; when the other person saw the flash, he uncovered his too. But Galileo's experimental distance wasn't far enough for his participants to record the speed of light. He could only conclude that light traveled at least 10 times faster than sound.
In the 1670s, Danish astronomer Ole Rømer tried to create a reliable timetable for sailors at sea, and according to NASA , accidentally came up with a new best estimate for the speed of light. To create an astronomical clock, he recorded the precise timing of the eclipses of Jupiter's moon , Io, from Earth . Over time, Rømer observed that Io's eclipses often differed from his calculations. He noticed that the eclipses appeared to lag the most when Jupiter and Earth were moving away from one another, showed up ahead of time when the planets were approaching and occurred on schedule when the planets were at their closest or farthest points. This observation demonstrated what we today know as the Doppler effect, the change in frequency of light or sound emitted by a moving object that in the astronomical world manifests as the so-called redshift , the shift towards "redder", longer wavelengths in objects speeding away from us. In a leap of intuition, Rømer determined that light was taking measurable time to travel from Io to Earth.
Rømer used his observations to estimate the speed of light. Since the size of the solar system and Earth's orbit wasn't yet accurately known, argued a 1998 paper in the American Journal of Physics , he was a bit off. But at last, scientists had a number to work with. Rømer's calculation put the speed of light at about 124,000 miles per second (200,000 km/s).
In 1728, English physicist James Bradley based a new set of calculations on the change in the apparent position of stars caused by Earth's travels around the sun. He estimated the speed of light at 185,000 miles per second (301,000 km/s) — accurate to within about 1% of the real value, according to the American Physical Society .
Two new attempts in the mid-1800s brought the problem back to Earth. French physicist Hippolyte Fizeau set a beam of light on a rapidly rotating toothed wheel, with a mirror set up 5 miles (8 km) away to reflect it back to its source. Varying the speed of the wheel allowed Fizeau to calculate how long it took for the light to travel out of the hole, to the adjacent mirror, and back through the gap. Another French physicist, Leon Foucault, used a rotating mirror rather than a wheel to perform essentially the same experiment. The two independent methods each came within about 1,000 miles per second (1,609 km/s) of the speed of light.
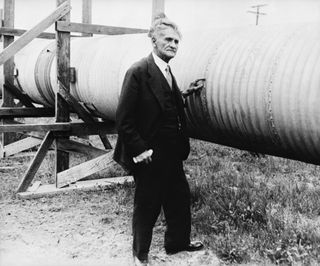
Another scientist who tackled the speed of light mystery was Poland-born Albert A. Michelson, who grew up in California during the state's gold rush period, and honed his interest in physics while attending the U.S. Naval Academy, according to the University of Virginia . In 1879, he attempted to replicate Foucault's method of determining the speed of light, but Michelson increased the distance between mirrors and used extremely high-quality mirrors and lenses. Michelson's result of 186,355 miles per second (299,910 km/s) was accepted as the most accurate measurement of the speed of light for 40 years, until Michelson re-measured it himself. In his second round of experiments, Michelson flashed lights between two mountain tops with carefully measured distances to get a more precise estimate. And in his third attempt just before his death in 1931, according to the Smithsonian's Air and Space magazine, he built a mile-long depressurized tube of corrugated steel pipe. The pipe simulated a near-vacuum that would remove any effect of air on light speed for an even finer measurement, which in the end was just slightly lower than the accepted value of the speed of light today.
Michelson also studied the nature of light itself, wrote astrophysicist Ethan Siegal in the Forbes science blog, Starts With a Bang . The best minds in physics at the time of Michelson's experiments were divided: Was light a wave or a particle?
Michelson, along with his colleague Edward Morley, worked under the assumption that light moved as a wave, just like sound. And just as sound needs particles to move, Michelson and Morley and other physicists of the time reasoned, light must have some kind of medium to move through. This invisible, undetectable stuff was called the "luminiferous aether" (also known as "ether").
Though Michelson and Morley built a sophisticated interferometer (a very basic version of the instrument used today in LIGO facilities), Michelson could not find evidence of any kind of luminiferous aether whatsoever. Light, he determined, can and does travel through a vacuum.
"The experiment — and Michelson's body of work — was so revolutionary that he became the only person in history to have won a Nobel Prize for a very precise non-discovery of anything," Siegal wrote. "The experiment itself may have been a complete failure, but what we learned from it was a greater boon to humanity and our understanding of the universe than any success would have been!"
Special relativity and the speed of light
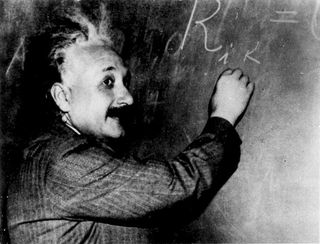
Einstein's theory of special relativity unified energy, matter and the speed of light in a famous equation: E = mc^2. The equation describes the relationship between mass and energy — small amounts of mass (m) contain, or are made up of, an inherently enormous amount of energy (E). (That's what makes nuclear bombs so powerful: They're converting mass into blasts of energy.) Because energy is equal to mass times the speed of light squared, the speed of light serves as a conversion factor, explaining exactly how much energy must be within matter. And because the speed of light is such a huge number, even small amounts of mass must equate to vast quantities of energy.
In order to accurately describe the universe, Einstein's elegant equation requires the speed of light to be an immutable constant. Einstein asserted that light moved through a vacuum, not any kind of luminiferous aether, and in such a way that it moved at the same speed no matter the speed of the observer.
Think of it like this: Observers sitting on a train could look at a train moving along a parallel track and think of its relative movement to themselves as zero. But observers moving nearly the speed of light would still perceive light as moving away from them at more than 670 million mph. (That's because moving really, really fast is one of the only confirmed methods of time travel — time actually slows down for those observers, who will age slower and perceive fewer moments than an observer moving slowly.)
In other words, Einstein proposed that the speed of light doesn't vary with the time or place that you measure it, or how fast you yourself are moving.
Therefore, objects with mass cannot ever reach the speed of light. If an object ever did reach the speed of light, its mass would become infinite. And as a result, the energy required to move the object would also become infinite: an impossibility.
That means if we base our understanding of physics on special relativity (which most modern physicists do), the speed of light is the immutable speed limit of our universe — the fastest that anything can travel.
What goes faster than the speed of light?
Although the speed of light is often referred to as the universe's speed limit, the universe actually expands even faster. The universe expands at a little more than 42 miles (68 kilometers) per second for each megaparsec of distance from the observer, wrote astrophysicist Paul Sutter in a previous article for Space.com . (A megaparsec is 3.26 million light-years — a really long way.)
In other words, a galaxy 1 megaparsec away appears to be traveling away from the Milky Way at a speed of 42 miles per second (68 km/s), while a galaxy two megaparsecs away recedes at nearly 86 miles per second (136 km/s), and so on.
"At some point, at some obscene distance, the speed tips over the scales and exceeds the speed of light, all from the natural, regular expansion of space," Sutter explained. "It seems like it should be illegal, doesn't it?"
Special relativity provides an absolute speed limit within the universe, according to Sutter, but Einstein's 1915 theory regarding general relativity allows different behavior when the physics you're examining are no longer "local."
"A galaxy on the far side of the universe? That's the domain of general relativity, and general relativity says: Who cares! That galaxy can have any speed it wants, as long as it stays way far away, and not up next to your face," Sutter wrote. "Special relativity doesn't care about the speed — superluminal or otherwise — of a distant galaxy. And neither should you."
Does light ever slow down?
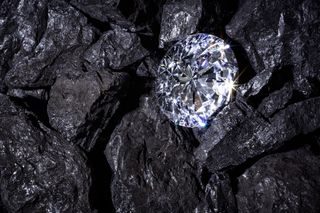
Light in a vacuum is generally held to travel at an absolute speed, but light traveling through any material can be slowed down. The amount that a material slows down light is called its refractive index. Light bends when coming into contact with particles, which results in a decrease in speed.
For example, light traveling through Earth's atmosphere moves almost as fast as light in a vacuum, slowing down by just three ten-thousandths of the speed of light. But light passing through a diamond slows to less than half its typical speed, PBS NOVA reported. Even so, it travels through the gem at over 277 million mph (almost 124,000 km/s) — enough to make a difference, but still incredibly fast.
Light can be trapped — and even stopped — inside ultra-cold clouds of atoms, according to a 2001 study published in the journal Nature . More recently, a 2018 study published in the journal Physical Review Letters proposed a new way to stop light in its tracks at "exceptional points," or places where two separate light emissions intersect and merge into one.
Researchers have also tried to slow down light even when it's traveling through a vacuum. A team of Scottish scientists successfully slowed down a single photon, or particle of light, even as it moved through a vacuum, as described in their 2015 study published in the journal Science . In their measurements, the difference between the slowed photon and a "regular" photon was just a few millionths of a meter, but it demonstrated that light in a vacuum can be slower than the official speed of light.
Can we travel faster than light?
— Spaceship could fly faster than light
— Here's what the speed of light looks like in slow motion
— Why is the speed of light the way it is?
Science fiction loves the idea of "warp speed." Faster-than-light travel makes countless sci-fi franchises possible, condensing the vast expanses of space and letting characters pop back and forth between star systems with ease.
But while faster-than-light travel isn't guaranteed impossible, we'd need to harness some pretty exotic physics to make it work. Luckily for sci-fi enthusiasts and theoretical physicists alike, there are lots of avenues to explore.
All we have to do is figure out how to not move ourselves — since special relativity would ensure we'd be long destroyed before we reached high enough speed — but instead, move the space around us. Easy, right?
One proposed idea involves a spaceship that could fold a space-time bubble around itself. Sounds great, both in theory and in fiction.
"If Captain Kirk were constrained to move at the speed of our fastest rockets, it would take him a hundred thousand years just to get to the next star system," said Seth Shostak, an astronomer at the Search for Extraterrestrial Intelligence (SETI) Institute in Mountain View, California, in a 2010 interview with Space.com's sister site LiveScience . "So science fiction has long postulated a way to beat the speed of light barrier so the story can move a little more quickly."
Without faster-than-light travel, any "Star Trek" (or "Star War," for that matter) would be impossible. If humanity is ever to reach the farthest — and constantly expanding — corners of our universe, it will be up to future physicists to boldly go where no one has gone before.
Additional resources
For more on the speed of light, check out this fun tool from Academo that lets you visualize how fast light can travel from any place on Earth to any other. If you’re more interested in other important numbers, get familiar with the universal constants that define standard systems of measurement around the world with the National Institute of Standards and Technology . And if you’d like more on the history of the speed of light, check out the book " Lightspeed: The Ghostly Aether and the Race to Measure the Speed of Light " (Oxford, 2019) by John C. H. Spence.
Aristotle. “On Sense and the Sensible.” The Internet Classics Archive, 350AD. http://classics.mit.edu/Aristotle/sense.2.2.html .
D’Alto, Nick. “The Pipeline That Measured the Speed of Light.” Smithsonian Magazine, January 2017. https://www.smithsonianmag.com/air-space-magazine/18_fm2017-oo-180961669/ .
Fowler, Michael. “Speed of Light.” Modern Physics. University of Virginia. Accessed January 13, 2022. https://galileo.phys.virginia.edu/classes/252/spedlite.html#Albert%20Abraham%20Michelson .
Giovannini, Daniel, Jacquiline Romero, Václav Potoček, Gergely Ferenczi, Fiona Speirits, Stephen M. Barnett, Daniele Faccio, and Miles J. Padgett. “Spatially Structured Photons That Travel in Free Space Slower than the Speed of Light.” Science, February 20, 2015. https://www.science.org/doi/abs/10.1126/science.aaa3035 .
Goldzak, Tamar, Alexei A. Mailybaev, and Nimrod Moiseyev. “Light Stops at Exceptional Points.” Physical Review Letters 120, no. 1 (January 3, 2018): 013901. https://doi.org/10.1103/PhysRevLett.120.013901 .
Hazen, Robert. “What Makes Diamond Sparkle?” PBS NOVA, January 31, 2000. https://www.pbs.org/wgbh/nova/article/diamond-science/ .
“How Long Is a Light-Year?” Glenn Learning Technologies Project, May 13, 2021. https://www.grc.nasa.gov/www/k-12/Numbers/Math/Mathematical_Thinking/how_long_is_a_light_year.htm .
American Physical Society News. “July 1849: Fizeau Publishes Results of Speed of Light Experiment,” July 2010. http://www.aps.org/publications/apsnews/201007/physicshistory.cfm .
Liu, Chien, Zachary Dutton, Cyrus H. Behroozi, and Lene Vestergaard Hau. “Observation of Coherent Optical Information Storage in an Atomic Medium Using Halted Light Pulses.” Nature 409, no. 6819 (January 2001): 490–93. https://doi.org/10.1038/35054017 .
NIST. “Meet the Constants.” October 12, 2018. https://www.nist.gov/si-redefinition/meet-constants .
Ouellette, Jennifer. “A Brief History of the Speed of Light.” PBS NOVA, February 27, 2015. https://www.pbs.org/wgbh/nova/article/brief-history-speed-light/ .
Shea, James H. “Ole Ro/Mer, the Speed of Light, the Apparent Period of Io, the Doppler Effect, and the Dynamics of Earth and Jupiter.” American Journal of Physics 66, no. 7 (July 1, 1998): 561–69. https://doi.org/10.1119/1.19020 .
Siegel, Ethan. “The Failed Experiment That Changed The World.” Forbes, April 21, 2017. https://www.forbes.com/sites/startswithabang/2017/04/21/the-failed-experiment-that-changed-the-world/ .
Stern, David. “Rømer and the Speed of Light,” October 17, 2016. https://pwg.gsfc.nasa.gov/stargaze/Sun4Adop1.htm .
Join our Space Forums to keep talking space on the latest missions, night sky and more! And if you have a news tip, correction or comment, let us know at: [email protected].
Get the Space.com Newsletter
Breaking space news, the latest updates on rocket launches, skywatching events and more!
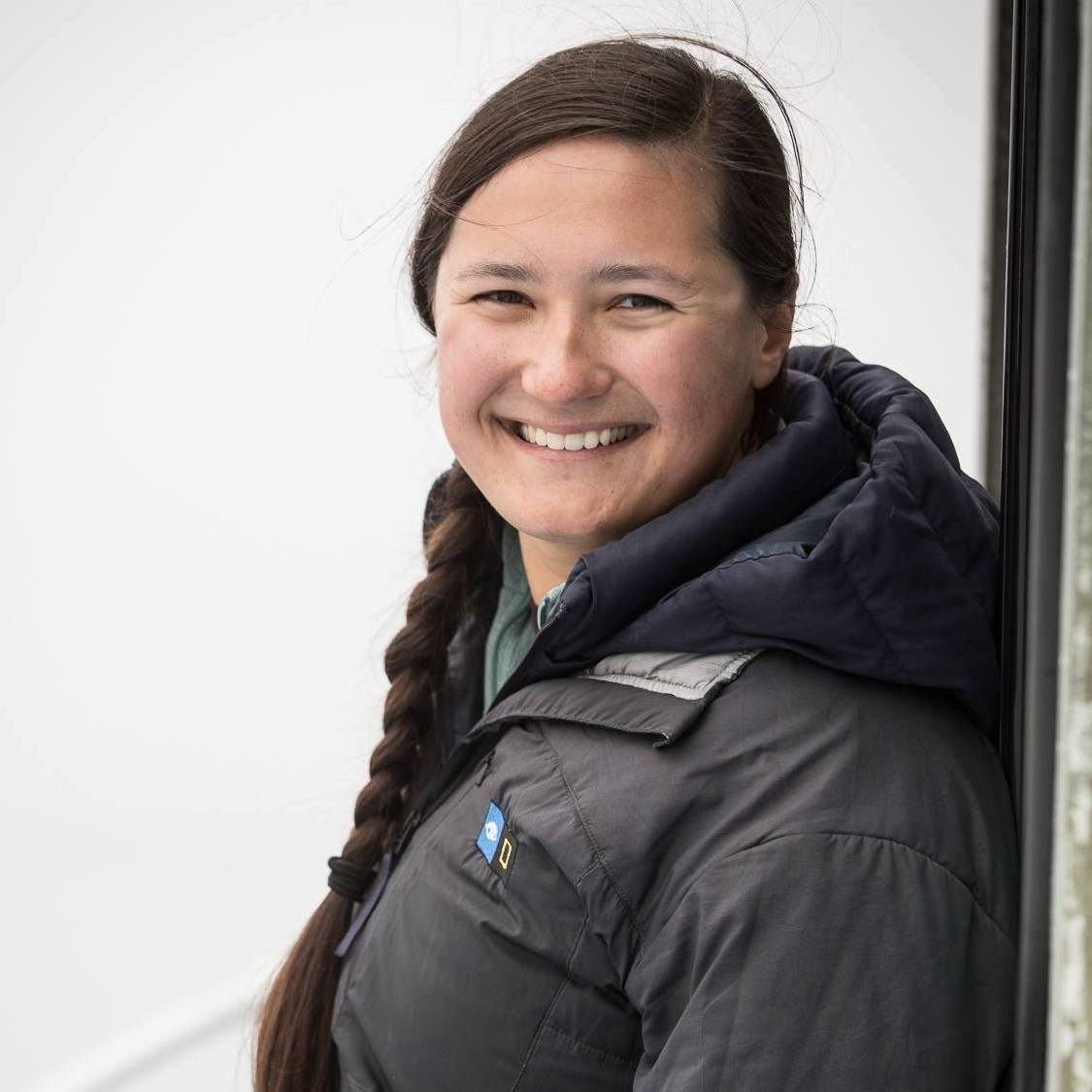
Vicky Stein is a science writer based in California. She has a bachelor's degree in ecology and evolutionary biology from Dartmouth College and a graduate certificate in science writing from the University of California, Santa Cruz (2018). Afterwards, she worked as a news assistant for PBS NewsHour, and now works as a freelancer covering anything from asteroids to zebras. Follow her most recent work (and most recent pictures of nudibranchs) on Twitter.
China's Chang'e 6 mission to collect samples of the far side of the moon enters lunar orbit (video)
China launches Chang'e 6 sample-return mission to moon's far side (video)
Netflix's asteroid-impact series 'Goodbye Earth' is an insufferably slow disaster saga (review)
Most Popular
- 2 Scientists use XRISM spacecraft to predict fate of matter around monster supermassive black hole
- 3 China's Chang'e 6 probe to the moon's far side has a big lunar mystery to solve
- 4 4 large incoming solar bursts could supercharge the auroras this weekend
- 5 Sierra Space's 1st Dream Chaser space plane aces key tests. Next stop: Florida launch site.
Want to create or adapt books like this? Learn more about how Pressbooks supports open publishing practices.
Traveling waves
17 How sound moves
Speed of sound.
There’s a delay between when a sound is created and when it is heard. In everyday life, the delay is usually too short to notice. However, the delay can be noticeable if the distance between source and detector is large enough. You see lightning before you hear the thunder. If you’ve sat in the outfield seats in a baseball stadium, you’ve experienced the delay between seeing the player hit the ball and the sound of the “whack.” Life experiences tell us that sound travels fast, but not nearly as fast as light does. Careful experiments confirm this idea.
The speed of sound in air is roughly 340 m/s. The actual value depends somewhat on the temperature and humidity. In everyday terms, sound travels about the length of three and a half foot ball fields every second- about 50% faster than a Boeing 747 (roughly 250 m/s). This may seem fast, but it’s tiny compared to light, which travels roughly a million times faster than sound (roughly 300,000,000 m/s).
Sound requires some material in which to propagate (i.e. travel). This material sound travels through is called the medium . You can show that sound requires a medium by putting a cell phone inside a glass jar connected to a vacuum pump. As the air is removed from the jar, the cell phone’s ringer gets quieter and quieter. A youTube video (2:05 min) produced by the UNSW PhysClips project shows the demo with a drumming toy monkey [1] instead of a cell phone.
What affects the speed of sound?
Sound travels at different speeds though different materials. The physical properties of the medium are the only factors that affect the speed of sound- nothing else matters.
The speed of sound in a material is determined mainly by two properties- the stiffness of the material and the density of the material. Sound travels fastest through materials that are stiff and light. In general, sound travels fastest through solids, slower through liquids and slowest through gasses. (See the table on this page). This may seem backwards- after all, metals are quite dense. However, the high density of metals is more than offset by far greater stiffness (compared to liquids and solids).
The speed of sound in air depends mainly on temperature. The speed of sound is 331 m/s in dry air at 0 o Celsius and increases slightly with temperature- about 0.6 m/s for every 1 o Celsius for temperatures commonly found on Earth. Though speed of sound in air also depends on humidity, the effect is tiny- sound travels only about 1 m/s faster in air with 100% humidity air at 20 o C than it does in completely dry air at the same temperature.
Nothing else matters
The properties of the medium are the only factors that affect the speed of sound- nothing else matters.
Frequency of the sound does not matter- high frequency sounds travel at the same speed as low frequency sounds. If you’ve ever listened to music, you’ve witnessed this- the low notes and the high notes that were made simultaneously reach you simultaneously, even if you are far from the stage. If you’ve heard someone shout from across a field, you’ve noticed that the entire shout sound (which contains many different frequencies at once) reaches you at the same time. If different frequencies traveled at different rates, some frequencies would arrive before others.
The amplitude of the sound does not matter- loud sounds and quiet ones travel at the same speed. Whisper or yell- it doesn’t matter. The sound still takes the same amount of time to reach the listener. You’ve probably heard that you can figure out how far away the lightning by counting the seconds between when you see lightning and hear thunder. If the speed of sound depended on loudness, this rule of thumb would have to account for loudness- yet there is nothing in the rule about loud vs. quiet thunder. The rule of thumb works the same for all thunder- regardless of loudness . That’s because the speed of sound doesn’t depend on amplitude.
Stop to thinks
- Which takes longer to cross a football field: the sound of a high pitched chirp emitted by a fruit bat or the (relatively) low pitched sound emitted by a trumpet?
- Which sound takes longer to travel 100 meters: the sound of a snapping twig in the forest or the sound of a gunshot?
- Which takes longer to travel the distance of a football field: the low pitched sound of a whale or the somewhat higher pitched sound of a human being?

Constant speed
Sound travels at a constant speed. Sound does not speed up or slow down as it travels (unless the properties of the material the sound is going through changes). I know what you’re thinking- how is that possible? Sounds die out as they travel, right? True. That means sounds must slow down and come to a stop, right? Wrong. As sound travels, its amplitude decreases- but that’s not the same thing as slowing down. Sound (in air) covers roughly 340 meters each and every second, even as its amplitude shrinks. Eventually, the amplitude gets small enough that the sound is undetectable. A sound’s amplitude shrinks as it travels, but its speed remains constant.
The basic equation for constant speed motion (shown below) applies to sound.
[latex]d=vt[/latex]
In this equation, [latex]d[/latex] represents the distance traveled by the sound, [latex]t[/latex] represents the amount of time it took to go that distance and [latex]v[/latex] represents the speed.
Rule of thumb for lightning example
Example: thunder and lightning.
The rule of thumb for figuring out how far away a lightning strike is from you is this:
Count the number of seconds between when you see the lightning and hear the thunder. Divide the number of seconds by five to find out how many miles away the lightning hit.
According to this rule, what is the speed of sound in air? How does the speed of sound implied by this rule compare to 340 m/s?
Identify important physics concept : This question is about speed of sound.
List known and unknown quantities (with letter names and units):
At first glance, it doesn’t look like there’s enough information to solve the problem. We were asked to find speed, but not given either a time or a distance. However, the problem does allow us to figure out a distance if we know the time- “Divide the number of seconds by five to find out how many miles away the lightning hit.” So, let’s make up a time and see what happens; if the time is 10 seconds, the rule of thumb says that the distance should be 2 miles.
[latex]v= \: ?[/latex]
[latex]d=2 \: miles[/latex]
[latex]t=10 \: seconds[/latex]
You might ask “Is making stuff up OK here?” The answer is YES! If the rule of thumb is right, it should work no matter what time we choose. (To check if the rule is OK, we should probably test it with more than just one distance-time combination, but we’ll assume the rule is OK for now).
Do the algebra: The equation is already solved for speed. Move on to the next step.
Do unit conversions (if needed) then plug in numbers: If you just plug in the numbers, the speed comes out in miles per second:
[latex]v = \frac{2 \: miles} {10 \: seconds}=0.2 \: \frac{miles} {second}[/latex]
We are asked to compare this speed to 340 m/s, so a unit conversion is in order; since there are 1609 meters in a mile:
[latex]v =0.2 \: \frac{miles} {second}*\frac{1609 \: meters} {1 \:mile}=320 \frac{m}{s}[/latex]
Reflect on the answer:
- The answer for speed from the rule of thumb is less than 10% off the actual value of roughly 340 m/s- surprisingly close!
- At the beginning, we assumed a time of 10 seconds. Does the result hold up for other choices? A quick check shows that it does! For instance, if we use a time of 5 seconds, the rule of thumb gives a distance of 1 mile, and the math still gives a speed of 0.2 miles/second. The speed will be the same no matter what time we pick. The reason is this: The more time it takes the thunder to arrive, the farther away the lightning strike is, but the speed remains the same. In the equation for speed, both time and distance change by the same factor and the overall fraction remains unchanged.
Stop to think answers
- Both sounds take the same amount of time. (High and low pitched sounds travel at the same speed).
- Both sounds take the same amount of time. (Quiet sounds and loud sounds travel at the same speed).
- The sound of the whale travels the distance in less time- assuming sound from the whale travels in water and sound from the human travels in air. Sound travels faster in water than in air. (The info about frequency was given just to throw you off- frequency doesn’t matter).
- Wolfe, J. (2014, February 20). Properties of Sound. Retrieved from https://www.youtube.com/watch?v=P8-govgAffY ↵
Understanding Sound Copyright © by dsa2gamba and abbottds is licensed under a Creative Commons Attribution 4.0 International License , except where otherwise noted.
Share This Book
Sciencing_Icons_Science SCIENCE
Sciencing_icons_biology biology, sciencing_icons_cells cells, sciencing_icons_molecular molecular, sciencing_icons_microorganisms microorganisms, sciencing_icons_genetics genetics, sciencing_icons_human body human body, sciencing_icons_ecology ecology, sciencing_icons_chemistry chemistry, sciencing_icons_atomic & molecular structure atomic & molecular structure, sciencing_icons_bonds bonds, sciencing_icons_reactions reactions, sciencing_icons_stoichiometry stoichiometry, sciencing_icons_solutions solutions, sciencing_icons_acids & bases acids & bases, sciencing_icons_thermodynamics thermodynamics, sciencing_icons_organic chemistry organic chemistry, sciencing_icons_physics physics, sciencing_icons_fundamentals-physics fundamentals, sciencing_icons_electronics electronics, sciencing_icons_waves waves, sciencing_icons_energy energy, sciencing_icons_fluid fluid, sciencing_icons_astronomy astronomy, sciencing_icons_geology geology, sciencing_icons_fundamentals-geology fundamentals, sciencing_icons_minerals & rocks minerals & rocks, sciencing_icons_earth scructure earth structure, sciencing_icons_fossils fossils, sciencing_icons_natural disasters natural disasters, sciencing_icons_nature nature, sciencing_icons_ecosystems ecosystems, sciencing_icons_environment environment, sciencing_icons_insects insects, sciencing_icons_plants & mushrooms plants & mushrooms, sciencing_icons_animals animals, sciencing_icons_math math, sciencing_icons_arithmetic arithmetic, sciencing_icons_addition & subtraction addition & subtraction, sciencing_icons_multiplication & division multiplication & division, sciencing_icons_decimals decimals, sciencing_icons_fractions fractions, sciencing_icons_conversions conversions, sciencing_icons_algebra algebra, sciencing_icons_working with units working with units, sciencing_icons_equations & expressions equations & expressions, sciencing_icons_ratios & proportions ratios & proportions, sciencing_icons_inequalities inequalities, sciencing_icons_exponents & logarithms exponents & logarithms, sciencing_icons_factorization factorization, sciencing_icons_functions functions, sciencing_icons_linear equations linear equations, sciencing_icons_graphs graphs, sciencing_icons_quadratics quadratics, sciencing_icons_polynomials polynomials, sciencing_icons_geometry geometry, sciencing_icons_fundamentals-geometry fundamentals, sciencing_icons_cartesian cartesian, sciencing_icons_circles circles, sciencing_icons_solids solids, sciencing_icons_trigonometry trigonometry, sciencing_icons_probability-statistics probability & statistics, sciencing_icons_mean-median-mode mean/median/mode, sciencing_icons_independent-dependent variables independent/dependent variables, sciencing_icons_deviation deviation, sciencing_icons_correlation correlation, sciencing_icons_sampling sampling, sciencing_icons_distributions distributions, sciencing_icons_probability probability, sciencing_icons_calculus calculus, sciencing_icons_differentiation-integration differentiation/integration, sciencing_icons_application application, sciencing_icons_projects projects, sciencing_icons_news news.
- Share Tweet Email Print
Mechanics Electricity & Magnetism Sound & Light Atomic & Nuclear Physics Heat Properties of Matter
Oscillations & waves.
- Home ⋅
- Science ⋅
Sound & Light (Physics): How are They Different?
Sound received by our human ears and light received by our human eyes may often seem to be two parts of the same phenomenon, but they are actually quite distinct.
While both phenomena are waves, their origins and properties vary considerably. By understanding these differences, we can make sense of our senses and gain a better handle on the underlying physics.
What Is a Wave?
A wave is a disturbance in a medium that propagates from one place to another via oscillations in that medium. The key here is that any point in the medium itself oscillates in place while the disturbance travels , transferring energy in the process.
Consider a crowd in a stadium doing the wave at a ball game. The members of the crowd are the wave medium. They oscillate in place by standing and sitting while the wave itself travels around the stadium.
Waves have wavelength, amplitude and frequency associated with them. The wavelength is the distance between successive wave peaks. The amplitude is the maximum displacement of the medium from equilibrium. The frequency is the number of wavelengths passing by a point per second.
Waves can be transverse or longitudinal. In a transverse wave, the medium oscillates perpendicularly to the direction of propagation. In a longitudinal wave, the medium oscillates along the same direction as propagation.
What Is a Sound Wave?
Sound is an example of a longitudinal wave. When a sound travels through a medium, such as air, it causes compressions (regions of increased density) and rarefactions (regions of decreased density) in the air as it travels.
Sound waves are created by oscillations – either from your vocal chords, a tuning fork, musical instruments or a pile of dishes crashing to the floor. A struck tuning fork, for example, vibrates at a specific frequency. As it moves, it bumps into the air molecules around it, periodically compressing them. The compressed regions transfer this energy to their neighboring air molecules as well, and the disturbance moves through the air.
What Are Light Waves?
Light, also called electromagnetic radiation (EM radiation) or electromagnetic waves, results from oscillations in electric and magnetic fields. If a charge moves back and forth along a wire, it creates a changing electric field, which in turn creates a changing magnetic field, which then self-propagates.
In this way, electromagnetic radiation serves as its own medium and does not require an external medium through which to travel, unlike most classical waves. Because of this, electromagnetic radiation can traverse the vacuum of space. (This is a good thing – otherwise light would never reach us from the sun!)
EM radiation comes in many different forms, depending on its wavelength and frequency, such as radio waves, microwaves, infrared radiation, visible light, ultraviolet light, X-rays and gamma rays. All of these waves travel at the speed of light in a vacuum (approximately 3 × 10 8 m/s). This is fastest speed in the universe! Faster-than-light travel is not physically possible.
How Are Radio Waves and EM Radiation Different From Sound Waves?
People often confuse these two types of waves because we are so familiar with listening to the radio through a speaker or headphones. And when it comes to orchestrating entertainment events, there are audio visual engineers needed.
But radio waves are a form of electromagnetic radiation. They travel at the speed of light, and they transmit information from the radio station to your radio. However, that information is then converted into the motion of a speaker, which produces sound waves, which are longitudinal waves in the air.
The speed of sound is approximately 343 m/s in air, which is much slower than radio waves, and they require a medium through which to travel.
Related Articles
- Georgia State University: HyperPhysics: Sound and Hearing
- The Physics Hypertextbook: The Nature of Light
About the Author
Gayle Towell is a freelance writer and editor living in Oregon. She earned masters degrees in both mathematics and physics from the University of Oregon after completing a double major at Smith College, and has spent over a decade teaching these subjects to college students. Also a prolific writer of fiction, and founder of Microfiction Monday Magazine, you can learn more about Gayle at gtowell.com.
Find Your Next Great Science Fair Project! GO
It’s a wonderful world — and universe — out there.
Come explore with us!
Science News Explores
Explainer: understanding waves and wavelengths.
Waves transfer energy, not matter, from one place to another
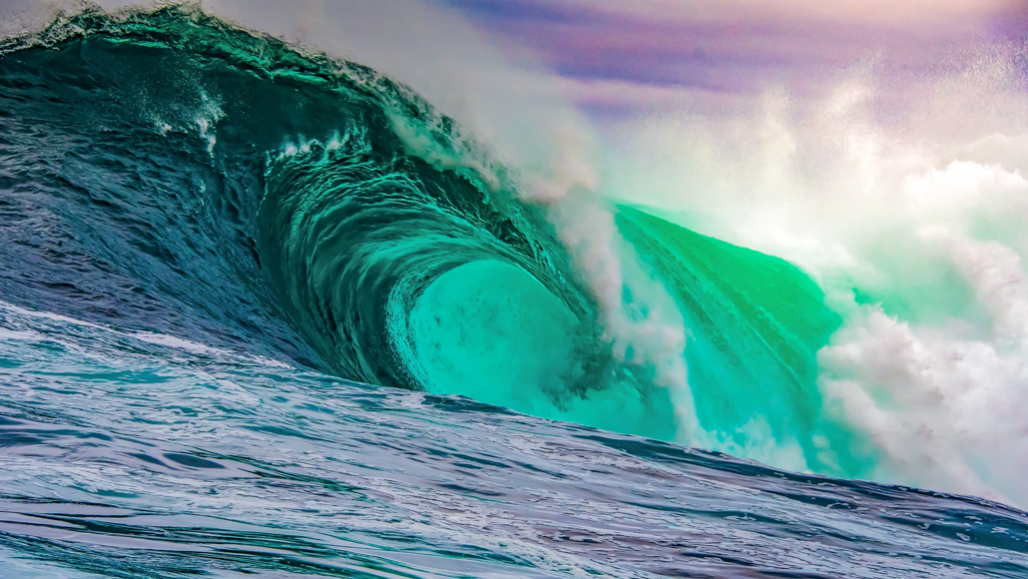
The swells of water in the ocean, the sunlight shining down and the sound of the crashing water ⎯ all are types of waves.
JNEphotos/iStock/Getty Images Plus
Share this:
- Google Classroom
By Jennifer Look
March 5, 2020 at 6:40 am
Waves appear in many different forms. Seismic waves shake the ground during earthquakes. Light waves travel across the universe, allowing us to see distant stars. And every sound we hear is a wave. So what do all these different waves have in common?
A wave is a disturbance that moves energy from one place to another. Only energy — not matter — is transferred as a wave moves.
The substance that a wave moves through is called the medium . That medium moves back and forth repeatedly, returning to its original position. But the wave travels along the medium. It does not stay in one place.
Imagine holding one end of a piece of rope. If you shake it up and down, you create a wave, with the rope as your medium. When your hand moves up, you create a high point, or crest. As your hand moves down, you create a low point, or trough (TRAWF). The piece of rope touching your hand doesn’t move away from your hand. But the crests and troughs do move away from your hand as the wave travels along the rope.
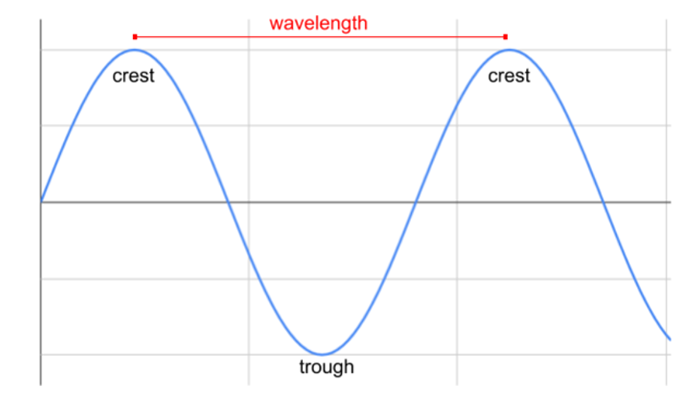
The same thing happens in other waves. If you jump in a puddle, your foot pushes on the water in one spot. This starts a small wave. The water that your foot hits moves outward, pushing on the water nearby. This movement creates empty space near your foot, pulling water back inwards. The water oscillates, moving back and forth, creating crests and troughs. The wave then ripples across the puddle. The water that splashes at the edge is a different bit of water than where your foot made contact. The energy from your jump moved across the puddle, but the matter (the molecules of water) only rocked back and forth.
Light, or electromagnetic radiation, also can be described as a wave. The energy of light travels through a medium called an electromagnetic field. This field exists everywhere in the universe. It oscillates when energy disturbs it, just like the rope moves up and down as someone shakes it. Unlike a wave in water or a sound wave in air, light waves don’t need a physical substance to travel through. They can cross empty space because their medium does not involve physical matter.
Scientists use several properties to measure and describe all these types of waves. Wavelength is the distance from one point on a wave to an identical point on the next, such as from crest to crest or from trough to trough. Waves can come in a wide range of lengths. The wavelength for an ocean wave might be around 120 meters (394 feet). But a typical microwave oven generates waves just 0.12 meter (5 inches) long. Visible light and some other types of electromagnetic radiation have far tinier wavelengths.
Frequency describes how many waves pass one point during one second. The units for frequency are hertz . Traveling through the air, a music note with a frequency of 261.6 hertz (middle C) pushes air molecules back and forth 261.6 times every second.
Frequency and wavelength are related to the amount of energy a wave has. For example, when making waves on a rope, it takes more energy to make a higher frequency wave. Moving your hand up and down 10 times per second (10 hertz) requires more energy than moving your hand only once per second (1 hertz). And those 10 hertz waves on the rope have a shorter wavelength than ones at 1 hertz.
Many researchers rely on the properties and behavior of waves for their work. That includes astronomers, geologists and sound engineers. For example, scientists can use tools that capture reflected sound, light or radio waves to map places or objects.
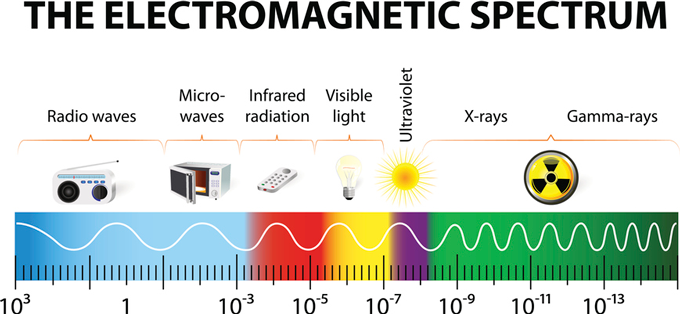
More Stories from Science News Explores on Physics
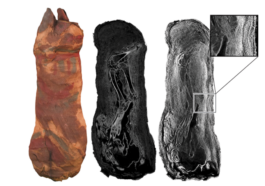
Let’s learn about particles that help us peer inside objects
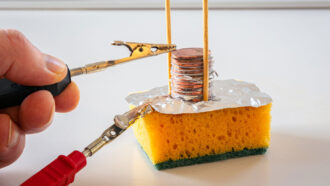
Experiment: Make your own cents-able battery
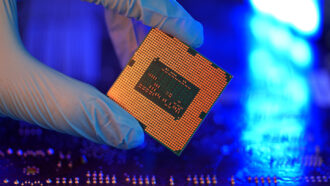
Scientists Say: Semiconductor
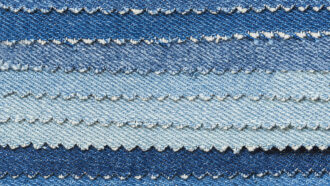
Turning jeans blue with sunlight might help the environment
Explainer: what is the solar cycle.
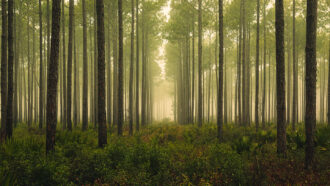
Forests could help detect ‘ghost particles’ from space
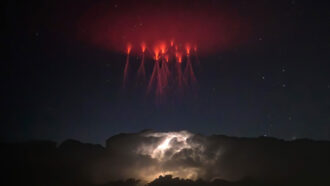
Explainer: Sprites, jets, ELVES and other storm-powered lights
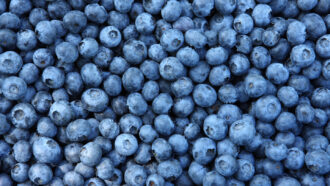
Here’s why blueberries aren’t blue — but appear to be
Does Light Travel Forever?
Most recent answer: 01/23/2013
Hi Raja, Good question. First, let's think about why sound does not travel forever. Sound cannot travel through empty space; it is carried by vibrations in a material, or medium (like air, steel, water, wood, etc). As the particles in the medium vibrate, energy is lost to heat, viscous processes, and molecular motion. So, the sound wave gets smaller and smaller until it disappears. In contrast, light waves can travel through a vacuum, and do not require a medium. In empty space, the wave does not dissipate (grow smaller) no matter how far it travels, because the wave is not interacting with anything else. This is why light from distant stars can travel through space for billions of light-years and still reach us on earth. However, light can also travel within some materials, like glass and water. In this case, some light is absorbed and lost as heat, just like sound. So, underwater, or in our atmosphere, light will only travel some finite range (which is different depending on the properties of the material it travels through). There is one more aspect of wave travel to consider, which applies to both sound and light waves. As a wave travels from a source, it propagates outward in all directions. Therefore, it fills a space given approximately by the surface area of a sphere. This area increases by the square of the distance R from the source; since the wave fills up all this space, its intensity decreases by R squared. This effect just means that the light/sound source will appear dimmer if we are farther away from it, since we don't collect all the light it emits. For example, light from a distant star travels outward in a giant sphere. Only one tiny patch of this sphere of light actually hits our eyes, which is why stars don't blind us! David Schmid
(published on 01/23/2013)
Follow-Up #1: How far does light go?
Light just keeps going and going until it bumps into something. Then it can either be reflected or absorbed. Astronomers have detected some light that has been traveling for more that 12 billion years, close to the age of the universe.
Light has some interesting properties. It comes in lumps called photons. These photons carry energy and momentum in specific amounts related to the color of the light. There is much to learned about light. I suggest you log in to our website and type LIGHT into the search box. Lots of interesting stuff there.
To answer your previous question "Can light go into a black hole?" , the answer is yes.
(published on 12/03/2015)
Follow-Up #2: less than one photon?
Certainly you can run the ouput of a single-photon source through a half-silvered mirror, and get a sort of half-ghost of the photon in two places. If you put ordinary photon detectors in those places, however, each will either detect zero or one. For each source photon, you'll get at most one of the detectors to find it. How does the half-ghost at the other one know whether it's detectably there or not? The name of that mystery is "quantum entanglement". At some level we don't really know the answer.
(published on 02/04/2016)
Follow-Up #3: stars too far away to see?
Most stars are too far for us to see them as individual stars even with our best telescopes. Still, we can get light from them, mixed with light from other stars. If our understanding of the universe is at all right, there are also stars that once were visible from here but now are outside our horizon so no light from them reaches us. It's probable that there are many more stars outside our horizon than inside, maybe infinitely more. It's hard to check, however, what's happening outside our horizon! It's even hard to define what we mean by "now" for things outside the horizon.
(published on 07/22/2016)
Follow-Up #4: light going out to space
Certainly ordinary light travels out to space. That's how spy cameras and such can take pictures of things here on the Earth's surface.
(published on 09/01/2016)
Follow-Up #5: end of the universe?
We don't think there's any "end" in the sense of some spatial boundary. Unless something changes drastically, there also won't be an end in time. The expansion looks like it will go on forever. So that wouldn't give a maximum range.
(published on 03/26/2017)
Follow-Up #6: seeing black holes
In principle a well-aimed beam would loop around the outside of the black hole and return to Earth. There aren't any black holes close enough to make this practical. Instead the bending of light by black holes is observed by their lensing effect on light coming from more distant objects.
The amazing gravitational wave signals observed from merging black holes provide even more direct and convincing proof that black holes exist and follow the laws of General Relativity.
(published on 01/29/2018)
Follow-up on this answer
Related Questions
- Can you use light to attract or repel an item?
- Absorption of short light pulses
- light from Hiroshima
- light dependent switches
- Would a tin-can phone work in space?
- refraction and reflection
- light reflection from glass
- light from old sources
- Seeing reflected and emitted light
- Speed of light in various directions
Still Curious?
Expore Q&As in related categories
- Properties of Light
- Properties of Sound
- Logout Login
- Adventure Holidays
- Weekend Getaways
- Driving Holidays
- Travel News
Iconic locations in Delhi to see light and sound shows
TRAVEL TRENDS , DELHI Created : May 2, 2024, 09:00 IST
Delhi, the capital city of India, is a treasure trove of history, culture, and architectural marvels. While the city's landmarks are captivating during the day, they take on a mesmerising charm after dark with spectacular light and sound shows. From ancient forts to majestic monuments, here are some must-visit places in Delhi for an enchanting evening of light and sound.
Step back in time and witness the grandeur of the Mughal era with the light and sound show at the iconic Red Fort. Held in the sprawling grounds of the fort, the show narrates the history of Delhi and the saga of Mughal emperors through captivating visuals, music, and narration. The illuminated ramparts of the Red Fort provide a stunning backdrop for this immersive experience, transporting visitors to a bygone era of splendour and intrigue.
Old Fort (Purana Qila)
Situated in lush greenery near the heart of the city, Old Fort is another historic site that comes alive at night with a mesmerising light and sound show. As dusk falls, the ancient walls of the fort are bathed in colourful lights, setting the stage for an enchanting journey through Delhi's rich past. The show highlights key events and legends associated with the fort, offering visitors a captivating glimpse into India's cultural heritage.
Akshardham Temple
One of Delhi's most magnificent modern marvels, Akshardham Temple dazzles visitors with its exquisite architecture and spiritual ambiance. In the evening, the temple complex is adorned with intricate lighting arrangements that accentuate its beauty and splendour. The light and sound show, titled ‘The Saga of Swaminarayan,’ unfolds against the backdrop of the temple's stunning facade, recounting the life and teachings of Swaminarayan through a captivating multimedia presentation.
Qutub Minar
Delhi's towering Qutub Minar, a UNESCO World Heritage Site, is another iconic monument that offers a captivating light and sound show experience. Set against the backdrop of the ancient minaret and the surrounding ruins, the show takes spectators on a journey through time, unravelling the mysteries and legends associated with Qutub Minar and its architectural marvels.
Visual Stories
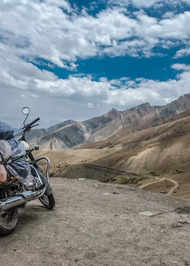
India's summer heavens: Top destinations for a refreshing vacation
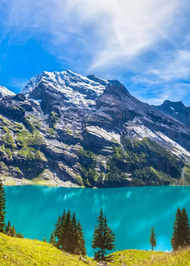
10 most economically stable countries in the world in 2024
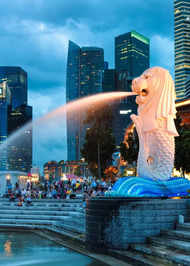
10 best cities in the world for curious travellers
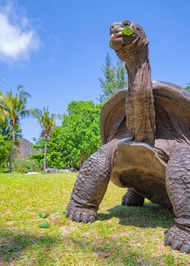
Oldest animal species alive on earth and where to spot them?
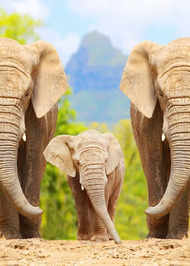
9 must-have travel experiences before you die!
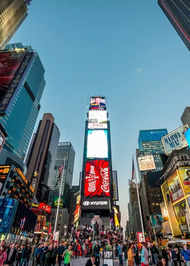
Top 10 largest cities in the world
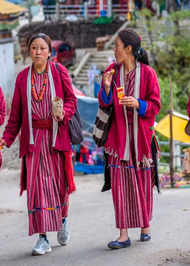
Indian destinations where temperature rarely rises above 20°C, even in summers!
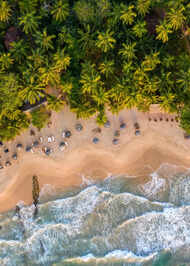
10 best visa-free beach destinations for Indians
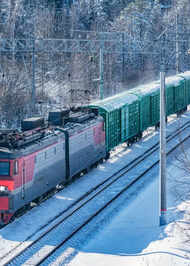
Biggest countries in the world by area
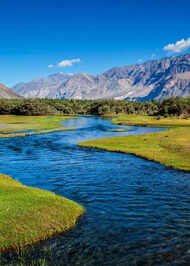
8 coldest places in India to escape the scorching heat
Join Us On Facebook Close
Poll of the day, which of these is one of earth's oldest geographical feature, comments (0).
Refrain from posting comments that are obscene, defamatory or inflammatory, and do not indulge in personal attacks, name calling or inciting hatred against any community. Help us delete comments that do not follow these guidelines by marking them offensive . Let's work together to keep the conversation civil.
Comments ( ) Sort: Newest UpVoted Oldest Discussed Down Voted closecomments
SIGN IN WITH
Or post without registration, trending stories.
Kedarnath Dham reopens for devotees on Akshay Tritiya; 6 things to know
Glass bridges in India worth travelling for
7 least populated cities in the world for an offbeat travel experience!
Israel announces it’s now open for tourism, and is ‘completely safe’
8 stunningly beautiful mountains in India
- Cool holiday ideas for all ages this summer
Top 10 smallest countries in the world
Thailand shuts down Pling Island due to extensive coral bleaching discovery
Venezuela becomes the first nation to lose all of its glaciers
From around the web, popular galleries.
In pictures: Most beautiful beaches in Kerala
Follow us on
Latest news.
- 5 must-have experiences in Nainital this summer
Congratulations!
You have been successfully added to the mailing list of Times of India Travel. To complete the subscription process, kindly open your inbox and click on the confirmation link which has been emailed to you.
Share with friends
Thank You for sharing! Your friend will receive the article link on email mentioned.
- (For more than one recipient, type addresses separated by commas)
Delhi, the capital city of India, is a treasure trove of history, culture, and architectural marvels. While the city's landmarks are captivating during the day, they take on a mesmerising charm after ...
If you're seeing this message, it means we're having trouble loading external resources on our website.
If you're behind a web filter, please make sure that the domains *.kastatic.org and *.kasandbox.org are unblocked.
To log in and use all the features of Khan Academy, please enable JavaScript in your browser.
Physics library
Course: physics library > unit 14, light: electromagnetic waves, the electromagnetic spectrum and photons.
- Electromagnetic waves and the electromagnetic spectrum
- Polarization of light, linear and circular
Introduction to electromagnetic waves
Basic properties of waves: amplitude, wavelength, and frequency, example: calculating the wavelength of a light wave, the electromagnetic spectrum, quantization of energy and the dual nature of light, example: calculating the energy of a photon, attributions.
- “ Electromagnetic Radiation ” from UC Davis ChemWiki, CC BY-NC-SA 3.0
Additional References
Want to join the conversation.
- Upvote Button navigates to signup page
- Downvote Button navigates to signup page
- Flag Button navigates to signup page

Search Icon
Events See all →
At-home anthro live.

1:00 p.m. - 1:45 p.m.
Alumni Weekend 2024

Various locations
268th Commencement

Franklin Field
Wawa Welcome America Day
10:00 a.m. - 5:00 p.m.
Penn Museum, 3260 South St.
Arts, Humanities, & Social Sciences
University-led research project seeks to streamline air travel
Megan ryerson of the weitzman school of design is part of a collaborative nasa-funded research team taming the turbulence of airport delays..
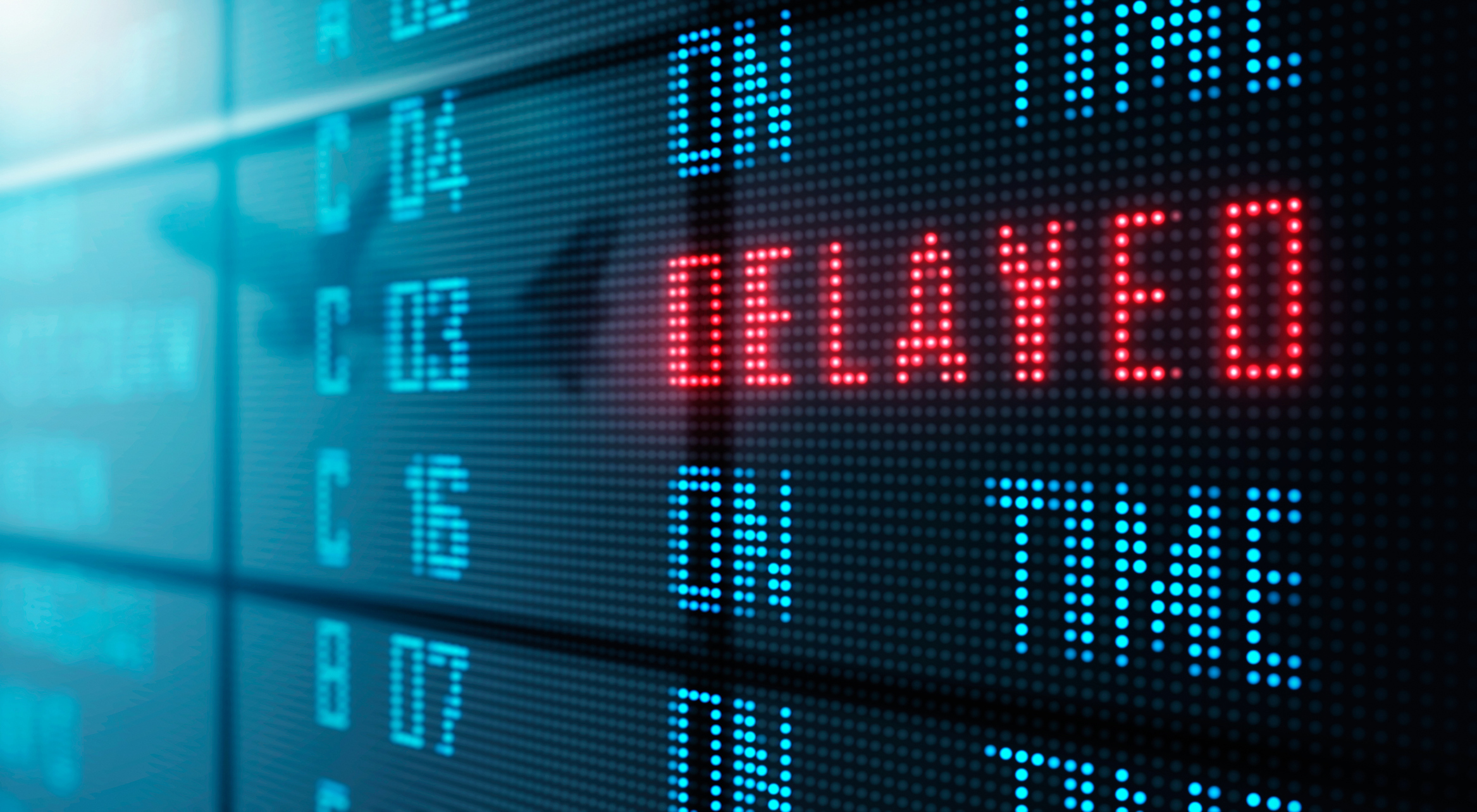
Long lines at the check-in counters, frustrated passengers stranded at airports, and the chaotic cancellation of flights due to unexpected storms or technical outages are becoming increasingly commonplace as more and more people rely on air travel, placing significant burden on decades-old infrastructure and protocols.
Responding to these growing challenges, Megan Ryerson , UPS Chair of Transportation at the University of Pennsylvania’s Weitzman School of Design and professor of city and regional planning and electrical and systems engineering at the School of Engineering and Applied Science , is part of a transformative research initiative aimed at bolstering the resilience of air travel. Funded by NASA, this project, led by a team of researchers and industry practitioners, will explore how the National Airspace System can be more adaptive to major storms, facility outages, and other technical issues that disrupt airline flight.
The project focuses on three critical thrusts aimed at transforming the National Airspace System: scenario generation; decision-making optimization; and validation, evaluation, and translation.
Scenario generation involves creating realistic simulations of potential issues such as weather disruptions and traffic congestions, allowing for proactive planning and robust contingency strategies.
The decision-making optimization portion uses advanced predictive models and operational research to provide recommendations for air traffic managers, ensuring that responses to any given scenario are effective and efficient.
And finally, the validation, evaluation, and translation focus ensures the practical application of research outcomes. It involves testing the proposed solutions in simulated environments and real-world settings to evaluate their impact and effectiveness, followed by formulating guidelines that can be implemented by stakeholders such as airport authorities, port authorities, the Federal Aviation Administration (FAA), and airlines.
The project, slated to begin in August, ironically came about during a discussion spurred by a major delay.
Turbulence at the TRB
Last year, on a chilly early January morning in Washington, D.C., Ryerson was attending the annual Transportation Research Board (TRB) conference.
Attendance ranges well into the thousands, Ryerson says, and it is a great way to connect and learn about what is going on in the field. “It’s the most special conference because it's a real motley crew of who’s who in transportation; there are a lot of Department of Transportation folks, a lot of practitioners, students, and your most seasoned professors,” she says.
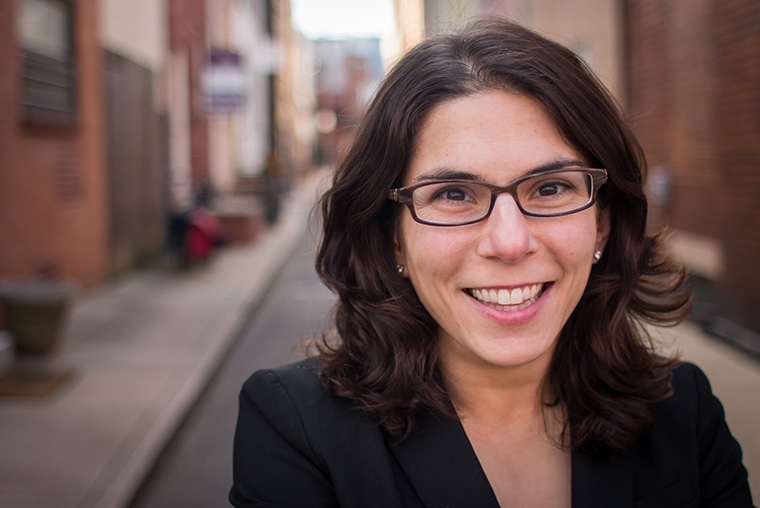
As the conference was winding down, “the Federal Aviation Administration experienced a significant outage in their Notice to Air Missions system (NOTAM), a critical piece of infrastructure that communicates necessary flight safety information to pilots," Ryerson says, “and, as you could imagine, passengers and airports were in disarray and flights were grounded across the nation.”
The following morning, amid the unfolding chaos, Ryerson had planned a breakfast with Dave Lovell, a professor at the University of Maryland and her longtime collaborator in air transportation optimization. As they headed to their meeting, “my phone buzzed relentlessly with updates and messages from my colleagues trapped at airports, texting me in disbelief at the extent of this disruption.”
Sitting down for breakfast at a café near the conference venue, the atmosphere was charged with a palpable sense of urgency, Ryerson says. “The catch-up session between me and Dave quickly turned into a NOTAM deep dive,” she says, “and, with our expertise in air transportation, we saw this event not as an isolated incident but as a symptom of the broader vulnerabilities within the air transportation system.”
They discussed how such disruptions were becoming more frequent and severe, exacerbated by factors like climate change and technological failures, and the conversation turned into a catalyst as the two looked into a way to bolster the resilience of air transportation. They aimed to develop strategies that could mitigate the effects of such disruptions and decided to formalize their discussions into a white paper focusing on resilience strategies and they planned to assemble a “dream team.”
This team would later include Max Li, a former student of Ryerson’s from Penn who is now an assistant professor of aerospace engineering at the University of Michigan; Patty Clark, as the former chief aviation strategic officer at the Port Authority of New York and New Jersey, with extensive experience monitoring and solving delay problems at major airports in the New York area; and Mark Hansen, a professor of civil engineering at the University of California, Berkeley, who had been Ryerson's Ph.D. advisor.
With the groundwork laid, Ryerson and her colleagues began reaching out to other potential collaborators and community colleges, involving them in crafting a full proposal to submit to NASA.
Expanded operations
By mid-2023, Ryerson and the team were ready to submit a comprehensive proposal. They targeted a specific NASA call for projects under the University Leadership Initiative and saw this as a unique opportunity to highlight the work of community colleges by including several neighboring institutions in their proposal.
“Education, outreach, and workforce development are a huge component of this project,” says Li, who will be serving as a faculty co-director in public outreach and supporting initiatives for spreading awareness of opportunities to learn more about the aerospace industry. “We’re here to highlight the broader roles that support the people we see when we hop on planes. From aircraft mechanics and engineers to airline and airport management there are programs from our partnered institutions that will help the next generation’s workforce join the bigger ecosystem here.”
Ryerson says that their work towards bolstering resilience within air transportation contributes to equity in mobility. “For many, paying that airfare is a big expense,” she says, we want to ensure “that, if the flight is cancelled because things happen, there are provisions in place to get you to your destination in a way that won’t negatively impact you or your finances.”
The nuts and bolts
Ryerson explains that the provisions she and her team seek to tie in some existing frameworks airlines use to remedy such problems, such as inter-airline agreements. “These are essentially deals that airlines may make with each other when they have to deal with stranded passenger during a disruption,” she says. “One airline will buy tickets from a competitor airline to accommodate passengers to make sure they can get stranded, disrupted passengers where they need to be.”
There are nuances, though, because companies want to make a profit, she says. “So strange things start to happen in this space. Like airline ‘X’ severing an inter-airline agreement with ‘Y,’ not selling to Y during an outage because X comes out looking a whole lot better if Y fails to deliver during a bad period.”
Ryerson also cites how airlines have years-long leases on gates at airports and that one airline may decide to block access to a gate from a competitor’s plane that needs to use it, which means “if your plane has to land at a gate that’s not permitted for use, and that’s the only one that’s open, you may just sit on the tarmac until a common-use gate opens up. Since there aren’t many common-use gates at most airports, that could be a long wait.”
Ryerson emphasizes the importance of predictive analytics and simulation-based preparedness in managing these inter-airline dynamics more effectively, and the team is taking the lessons learned to generate the next set of protocols.
Li's contributions to the proposal sheds light on the use of machine learning models to forecast air traffic scenarios and develop contingencies for each of the major stakeholders.
“For situation A, we’re going to have plan 1, plan 2, plan 3, and so on, and we’re going to make sure that these contingencies are tailored to whoever we’re talking to. For airlines, we adapt one set of models because their objectives are different than even another competitor airline. You could have different competing objectives and neither of these airlines’ objectives are necessarily aligned with that of the airport, which may not be aligned with that of the FAA. So, this is what makes the problem tricky because there are multiple stakeholders with multiple objectives, but that's what also makes it exciting from a mathematical perspective,” Li says.
“But you can make all the models you want and try predictions till you turn blue, but that means close to nothing if you don’t have a keen understanding of how situations on the ground tend to pan out, which is where Patty and her decades of experience at the airport have and continue to provide valuable insights,” Li says.
Connecting more than flights
Li and Ryerson note how Clark has helped them broaden their research beyond wheels-up to wheels-down to account for variables that often go unseen in the literature.
“Patty Clark is a brilliant leader in air transportation. Since the early days of my career, she has been teaching me about how the air transportation system truly runs and introducing all of us to parties involved from the Port Authority to get their perspectives. Patty is completely focused on passenger mobility and passenger customer service in air transportation,” Ryerson says.
“The Port Authority always took the position to make sure passengers are taken care of before and after they land, so in working with Mark, Megan, and more recently, Max, I had shared as much valuable information about the considerations staff make when things don’t go as planned,” Clark says. “If a plane is delayed, we need to assess the availability of services in and around the airport like concessions, taxis, rental cars, trains; we need to make sure there are personnel who can handle the cargo, unexpected baggage, or passengers with special needs, and so these are all considerations that may cascade if they’re not accounted for.”
Clark says she also works to keep the focus on the broader goals of air travel, citing President Harry S. Truman’s address at the grand opening of Idlewild Airport, now known as John F. Kennedy International Airport, in which he emphasized the importance of air travel in promoting international understanding and cooperation.
“He spoke about air travel not just as a means of transportation but as a gateway to peace and mutual respect among nations,” Clark says. “This vision of air travel as a bridge between cultures and communities is something that resonates deeply with our team's objectives.”
Clark explains that this historical perspective provides a framework for the team’s efforts today in improving the resilience and efficiency of air travel. “It’s not just about getting passengers from point A to point B. It’s about ensuring that air travel remains a reliable and accessible service that fosters connectivity and understanding across the globe,” she says.
Class of 2025 relishes time together at Hey Day
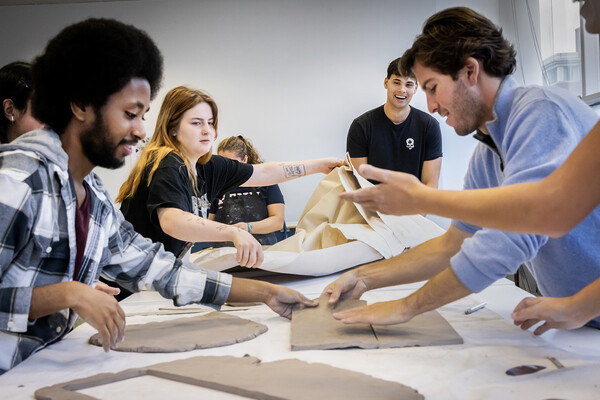
Picturing artistic pursuits
Hundreds of undergraduates take classes in the fine arts each semester, among them painting and drawing, ceramics and sculpture, printmaking and animation, photography and videography. The courses, through the School of Arts & Sciences and the Stuart Weitzman School of Design, give students the opportunity to immerse themselves in an art form in a collaborative way.
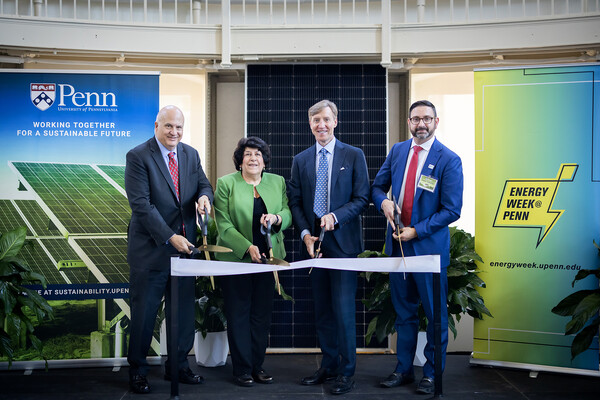
Campus & Community
Penn celebrates operation and benefits of largest solar power project in Pennsylvania
Solar production has begun at the Great Cove I and II facilities in central Pennsylvania, the equivalent of powering 70% of the electricity demand from Penn’s academic campus and health system in the Philadelphia area.
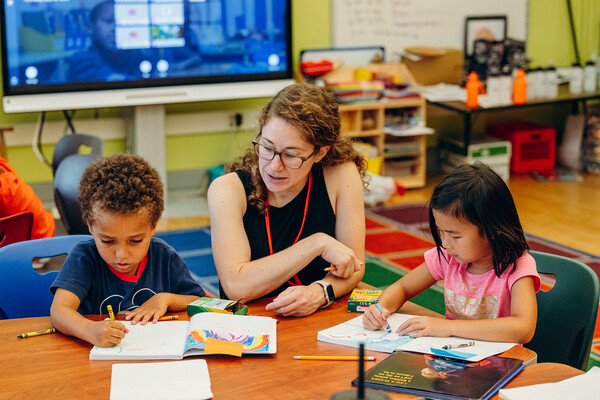
Education, Business, & Law
Investing in future teachers and educational leaders
The Empowerment Through Education Scholarship Program at Penn’s Graduate School of Education is helping to prepare and retain teachers and educational leaders.
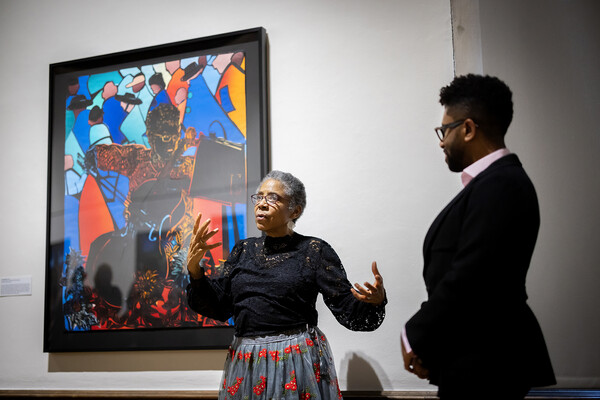
‘The Illuminated Body’ fuses color, light, and sound
A new Arthur Ross Gallery exhibition of work by artist Barbara Earl Thomas features cut-paper portraits reminiscent of stained glass and an immersive installation constructed with intricately cut material lit from behind.
You are using an outdated browser. Please upgrade your browser to improve your experience.
Find Posts By Topic
Travel advisory: president biden visiting seattle later this week – plan ahead and expect travel delays.

Blog stats: 400 words | 2-minute read
At-a-glance:
- President Joe Biden is scheduled to visit Seattle later this week.
- We expect significant travel delays and detours in the greater Seattle area in the coming days.
- Continue to check traffic conditions before you leave, and give yourself plenty of extra travel time.
- To stay informed, check King County Metro’s service advisories and sign up for transit alerts . You can also follow tweets from King County Metro , SDOT Traffic , and WSDOT Traffic .
- Thank you for your patience and cooperation during this Presidential visit to our region.
During President Biden’s visit, the U.S. Secret Service has the authority to provide security, including requiring intermittent closures of freeways and streets. The flow of buses and cars in downtown Seattle and around the region will be affected.
While the president’s exact travel route and timing are not published in advance, travelers can still plan ahead by anticipating delays and temporary road closures., what to expect and how to get around.
You can check media reports and the websites of local jurisdictions and transit agencies before you leave, so you have the latest information about travel conditions. Remember to give yourself plenty of extra time to reach your destination.
If you can, please consider options to get around without driving. Riding transit and biking can be good ways to avoid traffic, but please note that some bus routes may also be affected by temporary traffic closures or detours, including short-term re-routes.
Our Seattle Department of Transportation (SDOT) engineers can adjust traffic signal timing within the city of Seattle based on expected closures and any necessary re-routing. We may also post messages on dynamic message signs when needed.
How to stay informed
King County Metro publishes service advisories online and sends transit alerts to riders notifying them of bus re-routes and travel changes. You can see if your route is affected by visiting Metro’s service advisories page .
We also encourage you to sign up for Metro’s transit alerts and to monitor local news reports and social media updates.
- Sign up for King County Metro transit alerts
- Follow tweets from King County Metro , SDOT Traffic , and WSDOT Traffic .
Thank you again for your patience and cooperation during this Presidential visit.
Latest News
Sign up to receive updates from the SDOT Blog via email.
Browse the Archive
- Local Business
Eastside’s light rail expansion is good for the climate and the economy
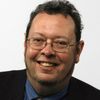
Sound Transit’s 2 Line opening last weekend on the Eastside drew about 17,500 people , confirming the promise of light rail.
For supporters, even the 6 miles completed of the eventual 14-mile corridor between Microsoft’s campus in Redmond, through Bellevue, and across Lake Washington to Seattle is progress.
Skeptics question spending $3.7 billion for the entire 14-mile, 10-station line to Seattle that might bring relatively small ridership. They note the botched construction on and around the floating bridge that pushed back completion of the line and worry whether crime on mass transit and the post-pandemic world of remote-or-hybrid work will make such a project worthwhile.
Although I hear complaints about safety on Link, my experiences have been uniformly safe and comfortable, certainly better than on buses. I recall a bumpy, uncomfortable bus ride from the University of Washington to downtown I frequently made before light rail. After that, it took me 10 minutes or less for the same journey.
My colleague Mike Lindblom has reported the situation in detail. In addition to the above issues, Link light rail has faced changing top leadership and grim downtown Seattle stations needing maintenance, sometimes forcing riders to contend with fentanyl users and rare (but resonating with the public) assaults .
The United States possesses around 27 light rail systems, more than any other nation. To be sure, similarly sized cities around the world often enjoy subways or other “heavy rail” mass transit on a scale far beyond that of America. Light rail operations carry fewer passengers compared with their heavy rail cousins.
As of this past year, San Diego, Los Angeles, Boston, Seattle and Portland showed the highest ridership. By contrast, L.A. offered the lengthiest system (95 miles), closely followed by “road warrior” Dallas, Philadelphia, San Diego and Portland (60 miles).
Link’s system runs a total of around 31 miles. When the system is completed in 2041 , it will total about 116 miles.
Seattle is still recovering from the mass transit system we might have won. In 1968 and 1970, a plan for a subway mostly funded by the federal government was defeated by voters.
Even modestly sized American cities once enjoyed rail transit in the form of streetcars and electric-powered interurban systems linking towns together, not to mention an abundance of commuter trains.
This faded most places with the rise in popularity of the automobile and the growth of car-dependent suburbia. The once-crowded streetcars were also doomed by cities forcing fares on the operators that were too low to keep the streetcars competitive. (The tale that General Motors wrecked the streetcars with its bus monopoly is largely considered a myth by transportation historians.)
In 2023, around 44% of federal infrastructure spending went to highways compared with only 22% for Amtrak and light rail. Public transportation in America has suffered a collapse in funding and commitment by government at all levels going back half a century.
I first encountered modern light rail when I lived in San Diego, where the San Diego Trolley debuted in 1981. At the time, it ran only from downtown to the Mexican border. I rode it and enjoyed the experience but failed to grasp its utility. Even so, as a young reporter I wrote about the system’s potential. Today it spans much of the metropolitan area on three different lines.
While I was working for the late, great Rocky Mountain News in Denver during the early 1990s, light rail again came into my consciousness. Initially, the Regional Transportation District opened a 5-mile segment downtown. Light rail was planned for Charlotte, N.C., when I lived there in the late 1990s (it opened in 2007).
Finally, I really became acquainted with the concept in Phoenix. Valley Metro, the regional transit agency, embarked on a starter line in 2008, running from the edge of Mesa, through Tempe and through downtown and Midtown Phoenix northwest for a total of 20 miles. It has since been extended far into Mesa and as far north as the now-defunct Metrocenter regional mall. As a columnist for The Arizona Republic, I supported light rail in the face of often hysterical opposition.
Such opposition is also found in supposedly “progressive” Seattle, where many voted against the Sound Transit initiatives and become incensed when I advocate building the First Avenue Streetcar.
But thanks to light rail, I haven’t been car burdened for 14 years. I don’t miss Happy Motoring.
Light rail can’t be seen without a connection to human-caused climate change. In Seattle, Link runs on hydropowered electricity, offering an option to greenhouse-gas-emitting automobiles.
The same can be said for the connection to economic development. For example, when the 2 Line is complete, it will offer tech employees an agreeable way to reach the Eastside offices of Amazon, Microsoft and numerous other Big Tech outposts and startups.
To be sure, light rail construction sometimes disrupts existing businesses — this generates big coverage in the media but the same is true for highway projects, which is mentioned less often. By contrast, when completed, light rail brings customers and offers opportunities for transit-oriented development near stations.
A partisan divide affects light rail as it does so much of America. Republicans generally are skeptical of this infrastructure, citing high costs and relatively low ridership. Democrats generally support it.
Most Read Business Stories
- Amazon founder Bezos unloads $8.5 billion in stock to lead Magnificent Seven insider sales
- Fundraiser for Molbak’s comeback bid falls short
- Can’t keep up with Seattle-area rising food prices? You’re not alone VIEW
- It’s now time to address the Boeing Problem or more turbulence awaits
- Whistleblower Josh Dean of Boeing supplier Spirit AeroSystems has died
But this isn’t always the case. In Oklahoma City, the heart of a red state, a 5-mile streetcar line opened downtown in 2018.
The success of light rail in the Puget Sound region will depend on keeping promises made to the voters, keeping trains safe and on time, as well as ensuring that projects come in as scheduled and on or under budget.
Editor’s note: This column has been updated to clarify the writer’s journey from the University of Washington to downtown.
The opinions expressed in reader comments are those of the author only and do not reflect the opinions of The Seattle Times.

IMAGES
VIDEO
COMMENTS
Sound is like light in some ways: it travels out from a definite source (such as an instrument or a noisy machine), just as light travels out from the Sun or a light bulb. But there are some very important differences between light and sound as well. We know light can travel through a vacuum because sunlight has to race through the vacuum of ...
The first notable difference between the speed of sound and light is how fast they are. In Earth's atmosphere, the speed of sound averages at about 761-miles per hour (1,225-kilometres per hour). That may seem fast, yet when compared to the speed of light, it seems quite small. Light travels at a staggering 670-million miles per hour (1.07 ...
Discover the physics behind the speed of light and sound, and how they affect our perception of the world around us.
Light and Sound. Light and sound are made of WAVES. They are both forms of ENERGY. Light travels much faster than sound. This is why the flash of lightening is seen long before the sound of thunder is heard, even though they are formed at the same instant. Speed of light 3×1010 m/s (300,000 km/s) Speed of sound 330m/s. Light.
Sound, like all waves, travels at certain speeds through different media and has the properties of frequency and wavelength. Sound travels much slower than light—you can observe this while watching a fireworks display (see Figure 14.6), since the flash of an explosion is seen before its sound is heard.
Sound travels as waves of energy, but, unlike light, the waves transmit energy by changing the motion of particles. Let's say you clap your hands together. ... The sound took 13 s to travel through the air, one group of particles hitting another, to get to the air particles that were near the spectators so that they could hear the sound.
In fact, we now define the speed of light to be a constant, with a precise speed of 299,792,458 meters per second. While it remains a remote possibility in deeply theoretical physics that light ...
Figure \(\PageIndex{1}\): When a firework explodes, the light energy is perceived before the sound energy. Sound travels more slowly than light does. (credit: Dominic Alves, Flickr) The relationship of the speed of sound, its frequency, and wavelength is the same as for all waves: \[v_w = f\lambda,\]
Light, on the other hand, is not a pressure wave—it's a fundamental particle. One ray of light is typically called a photon, and it's an electromagnetic disturbance. Light doesn't need a medium to travel. The speed of sound through air is about 340 meters per second. It's faster through water and it's even faster through steel.
Light travels much faster than sound. Light travels at a speed of 299,792,458 m/s (that's nearly 300,000 km/s!). The distance around the Earth is 40,000 km, so in 1 second, light could travel seven and a half times around the world. Sound only travels at about 330 m/s through the air, so light is nearly a million times faster than sound.
We can find the speed of sound by looking at the speed of this compressed region as it travels through the medium. In non-humid air at 20 degrees Celsius, the speed of sound is about 343 meters per second or 767 miles per hour. We can also watch the speed of sound of a repeating simple harmonic wave.
The speed of light traveling through a vacuum is exactly 299,792,458 meters (983,571,056 feet) per second. That's about 186,282 miles per second — a universal constant known in equations as "c ...
This may seem fast, but it's tiny compared to light, which travels roughly a million times faster than sound (roughly 300,000,000 m/s). The medium. Sound requires some material in which to propagate (i.e. travel). This material sound travels through is called the medium. You can show that sound requires a medium by putting a cell phone inside ...
The speed of light in vacuum, commonly denoted c, is a universal physical constant that is exactly equal to 299,792,458 metres per second (approximately 300,000 kilometres per second; 186,000 miles per second; 671 million miles per hour). According to the special theory of relativity, c is the upper limit for the speed at which conventional matter or energy (and thus any signal carrying ...
The speed of light is much faster than the speed of sound in air. If you want to compare, the speed of sound in air is ~ 343 m/s and the speed of light is 3x1010m/s. In other words, light travels 186 thousand miles in 1 second, while sound takes almost 5 seconds to travel 1 mile. (published on 02/14/2017)
For instance, if you heat up the air that a sound wave is travelling through, the density of the air decreases. This explains why sound travels faster through hotter air compared to colder air. The speed of sound at 20 degrees Celsius is about 343 meters per second, but the speed of sound at zero degrees Celsius is only about 331 meters per second.
A Ray of Light. When an electromagnetic source generates light, the light travels outward as a series of concentric spheres spaced in accordance with the vibration of the source. Light always takes the shortest path between a source and destination. A line drawn from the source to the destination, perpendicular to the wave-fronts, is called a ray.
The speed of light. The speed of light in air is very close to 300 000 000 m/s. which is nearly a million times faster than the speed of sound, which is 340 m/s. 300 000 000 m/s is often written ...
Sound received by our human ears and light received by our human eyes may often seem to be two parts of the same phenomenon, but they are actually quite distinct. Sound waves are longitudinal waves that can travel through air at about 343 m/s. Light waves are EM radiation and travel at 3 × 10^8 m/s.
The energy of light travels through a medium called an electromagnetic field. This field exists everywhere in the universe. It oscillates when energy disturbs it, just like the rope moves up and down as someone shakes it. Unlike a wave in water or a sound wave in air, light waves don't need a physical substance to travel through.
Sound cannot travel through empty space; it is carried by vibrations in a material, or medium (like air, steel, water, wood, etc). As the particles in the medium vibrate, energy is lost to heat, viscous processes, and molecular motion. So, the sound wave gets smaller and smaller until it disappears. In contrast, light waves can travel through a ...
Sound propagates through air or other mediums as a longitudinal wave, in which the mechanical vibration constituting the wave occurs along the direction of propagation of the wave. A longitudinal wave can be created in a coiled spring by squeezing several of the turns together to form a compression and then releasing them, allowing the compression to travel the length of the spring.
TRAVEL TRENDS, DELHI Created : May 2, 2024, 09:00 IST Iconic locations in Delhi to see light and sound shows Delhi, the capital city of India, is a treasure trove of history, culture, and ...
Electromagnetic radiation is one of the many ways that energy travels through space. The heat from a burning fire, the light from the sun, the X-rays used by your doctor, as well as the energy used to cook food in a microwave are all forms of electromagnetic radiation. While these forms of energy might seem quite different from one another ...
'The Illuminated Body' fuses color, light, and sound. A new Arthur Ross Gallery exhibition of work by artist Barbara Earl Thomas features cut-paper portraits reminiscent of stained glass and an immersive installation constructed with intricately cut material lit from behind.
We expect significant travel delays and detours in the greater Seattle area in the coming days. Continue to check traffic conditions before you leave, and give yourself plenty of extra travel time. To stay informed, check King County Metro's service advisories and sign up for transit alerts.
Sound Transit's 2 Line opening last weekend on the Eastside drew about 17,500 people, confirming the promise of light rail. For supporters, even the 6 miles completed of the eventual 14-mile ...